Blog
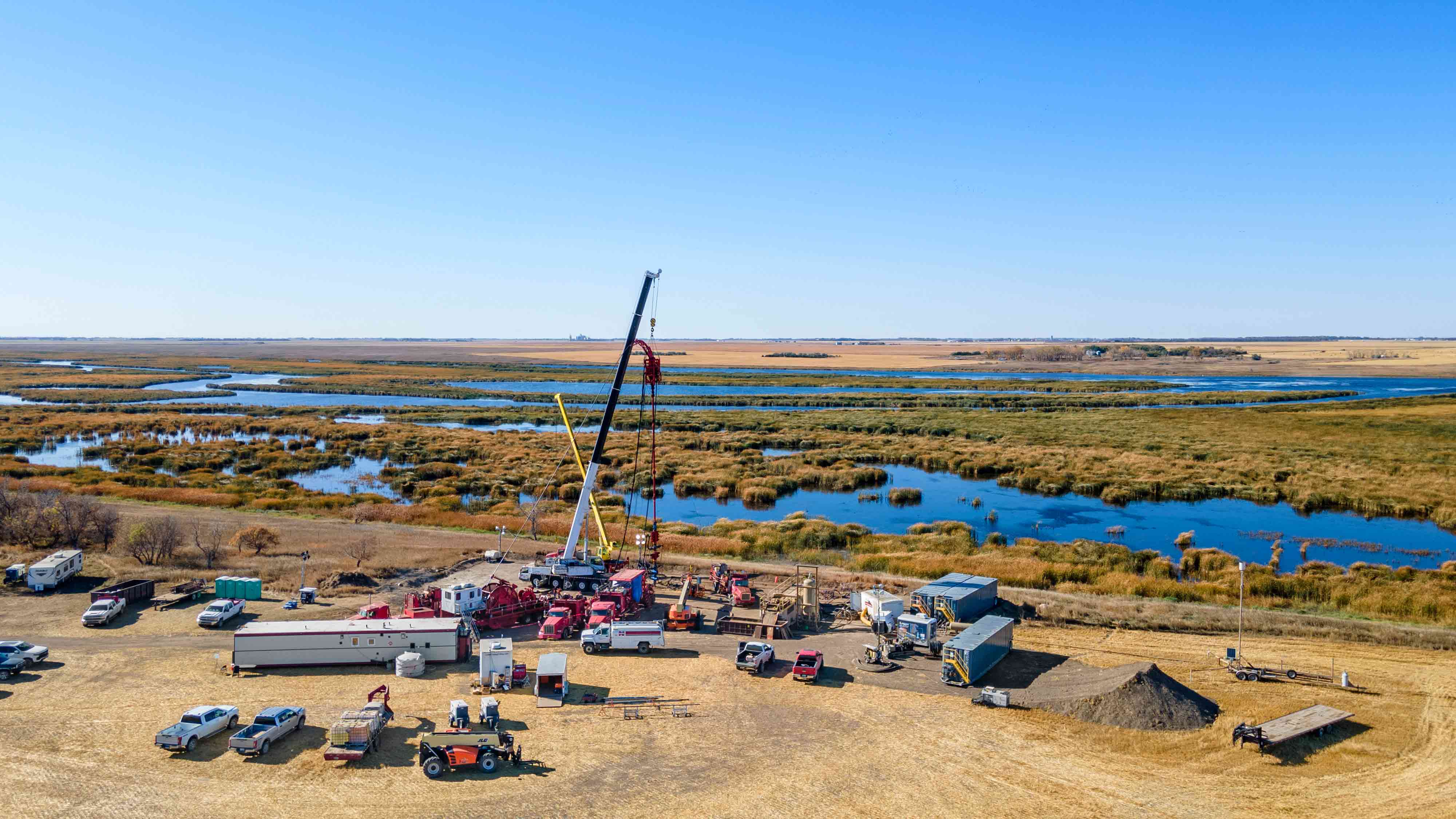
1705498542000
How To Achieve Step-By-Step Energy Transition
The energy sector is making a global effort to move away from the use of fossil fuels, instead turning towards a future of renewable energy sources such as wind, solar and geothermal energy, ahead of the 2050 net zero deadline. However, there is still a lot to be done in order to hit this target. One of humankind’s greatest challenges The UN has admitted that the world is currently not on track to hit these targets, stating that transitioning to net-zero is one of the ‘greatest challenges humankind is facing’. It stated that new, stronger, commitments are needed from governments and private companies to meet the 2050 deadline. For many, ripping and replacing existing energy sources is unrealistic. The challenge to achieve net-zero is a big one and unless there is a step-by-step process that allows governments and companies to make real strides towards a net zero future, whilst providing a halfway house, there could be a danger that heads will remain in the sand. Minerals and mining are required for a renewable energy future A renewable energy future does not mean one without the need for minerals and mining and this is a fact that is often overlooked. The demand for renewable energy means that more minerals will in fact be needed. Therefore, there will be a requirement for current technology solutions that collect minerals to remain in some form. Also, The London School of Economics and Political Science (LSE) believes that carbon capture, usage and storage can play a strategic role in global decarbonisation and can offer the most cost-effective option for many regions. These carbon reduction targets will mean an increased need for carbon capture and storage. According to the LSE report, any CO2 leakage could lead to environmental damage that reverses emissions savings. Drilling technology that already has carbon capture and storage capabilities will be crucial for the energy sector. The 2050 net-zero deadline does not mean the end of oil and gas. In fact they will make up as much as 20 percent of current levels. In order to make substantial changes to the way we use and gather energy, oil and gas will be very much needed to power the solutions that do this. Drilling will also remain in some form, although with a different focus. Companies that currently supply innovative solutions to the oil and gas drilling sector will be integral in helping to move the sector forward through the use of geothermal, mining and underground storage. Recycling current energy technology to reduce costs Cost is one of the main issues of the energy transition. Companies assume that current technologies will need to be completely replaced to hit targets, when actually current technology and solutions used within the energy sector are crucial for a net-zero future. Specialist drilling technology can reduce the cost of drilling wells for carbon storage, geothermal and mining. Technology currently utilised in the oil and gas sector will also be vital for containing hydrogen. Geothermal energy could help to create electricity. The difficulty lies in creating and maintaining the extraordinarily high-temperatures that are needed to produce it, thus making it financially impossible. Current high-temperature electronics used in the oil and gas industry can be used for geothermal wells. This helps to reduce additional investment, whilst allowing companies to use this ‘cleaner’ method of creating electricity. Overall, the oil and gas sector is able to ‘recycle’ current technology to help with the energy transition. Even though this seems counter-intuitive, it actually makes a great deal of sense. Indeed, technology providers to the sector are very much part of the solution rather than part of the problem. The key to success is collaboration Oil and gas will remain critical in a net-zero world, indeed the innovative technology will be crucial in allowing the sector and world to move towards a more sustainable future. The latest research all points to governments and organisations not making enough progress ahead of the 2050 deadline. However, this is not a quick fix, the energy mix will change over time, but it is not the right approach to do away with current methods just because they are associated with oil and gas. Collaboration will be crucial in order to hit deadlines a create a ‘cleaner’ environment. Current technologies and solutions are key to moving the sector forward, reducing costs and ensuring that new methods of creating energy can be brought on board and quickly utilised to create a more sustainable future. Author: Toni Miszewski
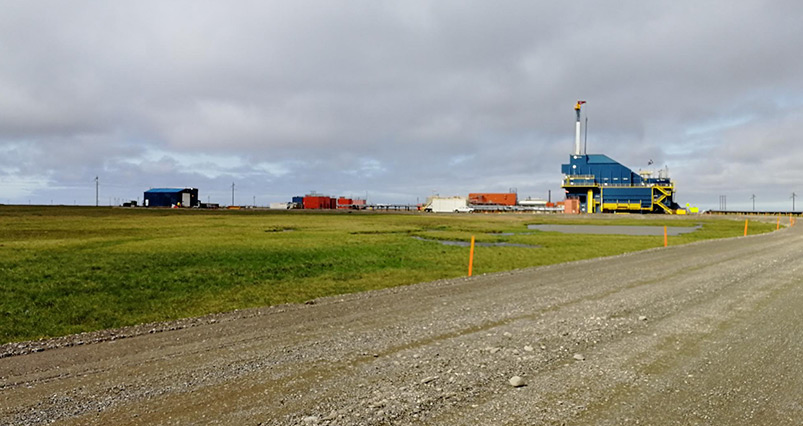
1600689600000
Case Study: Longest Lateral Drilled in 24hrs on the North Slope using at-balance CTD
Objectives and Challenges The two wells under consideration in this case study were injection wells. Extended reach laterals in a sand formation were planned with the primary objective being to support production from other wells in the field. Extended reach was critical for economic viability of the project with horizontal laterals exceeding beyond any length achieved previously. CTD was seen as the best way to drill the reservoir because of cost advantages as well as the ability to drill at-balance using managed pressure drilling to keep the wellbore at a stable bottom hole pressure. The key objective was to drill as far as possible helped by a very straight hole. Pre-Job Planning For the project to be economically viable, it was determined that laterals of over 6000ft were required. Tubing forces modelling was used to test the sensitivity of a variety of ways to increase lateral length. Various options were modelled and compared to a base case of 2-3/8” CT. These included the use of an extended reach tool, improving the friction factor in cased hole and open hole, increasing the tubing diameter and drilling a hole with reduced DLS. The relative effect of all of these options was compared and the largest improvement in reach is created by reducing the DLS of the drilled borehole. The straightness of the borehole is a function of the type of orienter used. The most advanced orienter on the market is a Continuous Rotation Orienter (CRO). Continuous rotation drills a straight hole by pointing the bit in all toolface angles as it rotates in the wellbore. So, even though the motor has a bend, it never settles in one unique direction. Older technology orienters do not have this capability so they need to approximate a straight hole by drilling a short way oriented to the right and then a short way oriented to the left. When this is done repeatedly, TVD is maintained but a wavy hole with varying azimuth is drilled with a DLS that approximates 7⁰/100ft. It also enables the build section and a straight tangent section of a well to be drilled in one run, without having to pull to surface to change the bend. This adaptable and precise control also leads to a smooth build section which also helps extend reach. Execution A triple casing exit was performed as planned for both wells by milling through the 4.5”, 7.0” and 9.625” strings with two milling runs required for each exit. After the triple window was milled, the hole was circulated clean and the BHA pulled to surface. The BHA was changed to a steerable drilling one and tripped back in to drill the build section. Tight directional control was maintained with the as-drilled trajectory closely matching the plan (Fig 1). This was maintained with drilling rates in the order of 180fph where an error in steering can quickly lead to significant deviations from the planned path. In the latter stages, friction reducer was added to the drilling fluid to improve WOB. Figure 1 Deviation from planned path Specific achievements These were the first triple casing exit performed with CT on the North Slope. Longest lateral section drilled in a 24hr period at 2894ft. Longest liner run in one run on coiled tubing. Author: Toni Miszewski
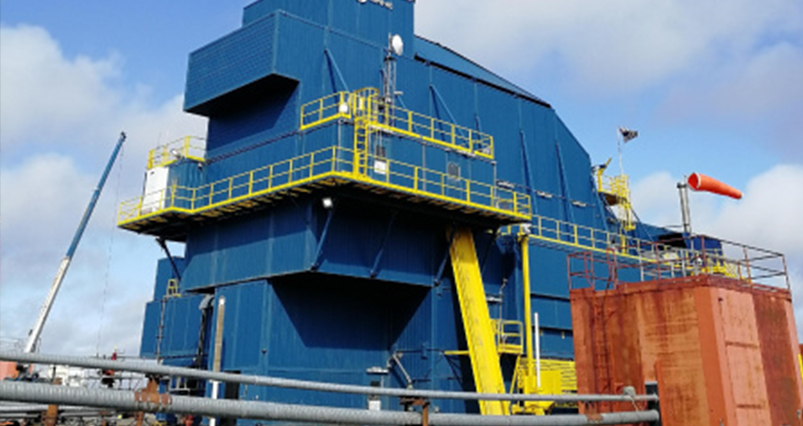
1594382400000
Case Study: Coiled Tubing Drilling, Kuparuk Oilfield, North Slope Alaska (SPE 168250)
North Slope Alaska can boast one of the largest numbers for wells drilled using Coiled Tubing Drilling technology. As redevelopment of the Alaska fields continues, horizontal limits are pushed to even greater maximums. Kuparuk oilfield is a mature field on the North Slope that features thin, highly faulted reservoirs, making the CTD an instrumental technology to drill horizontal multilateral thru-tubing completions. In October 2012, a well was drilled using MPD techniques. The well was a tri-lateral injection well drilled to the south to support a production well in the area. It was planned to drill an open hole section of 8143’, with 4318’ laterally. Laterals were all completed using a 2-3/8” slotted liner. There were several challenges associated with drilling this well, particularly, formation pressure management was more challenging due to absence of reliable data regarding the pressure in the southern-most fault blocks. The estimate was about 9.8 ppg but it was not possible to confirm this. The near wellbore pressure was about 12.6 ppg. Using these estimated values, an ECD operating envelope was constructed, which allowed the ability to determine the maximum permissible annular friction pressure. The borehole size had to be increased to 3.25” from 3.00” which was originally planned. The first lateral was a record-breaking length of 4,293 ft of openhole at a TD of 13,375 ft MD. Nonetheless, this was not without obstacles as heavy losses were encountered. The initial estimate of 9.8 ppg suggested that formation breakdown could happen above 13.8 ppg EMW. However, circulation was lost at just under 13.8 ppg, suggesting that the estimate should have been closer to 9.6 ppg. Another challenge was running the liner completion. Due to heavy fluid losses, the liner running pill was lost to the formation, increasing drag and causing the lock up at 13,028’ MD, 347’ short of TD. The operator re-assessed the drilling parameters before going onto the next two laterals to avoid similar complications. It was decided ECD would be lowered in the openhole by adjusting mud properties and pump rate. This modification resulted in zero losses when drilling the subsequent laterals. The laterals were drilled using a very low AKO on the drilling motor (about 0.5° AKO) with a bi-center 3.25” bit. Average doglegs were resulted in 6-8 deg/100ft. Even though, a cumulative dogleg was consistent with the directional plan (7° DLS), the actual drilled trajectory and the planned trajectory ended up being very different. Nonetheless, the project was successful with all laterals hitting target zones. This project expanded capabilities of extended reach lateral drilling on Coiled Tubing and provided additional insight to areas that required further development and work. Author: Marat Seitimov
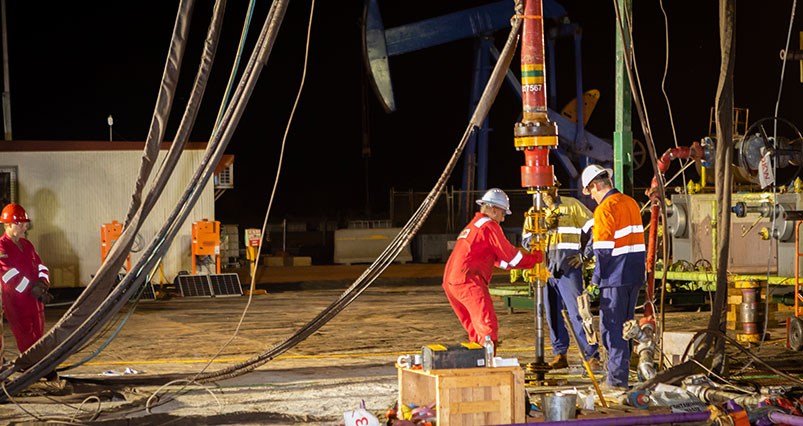
1590494400000
Case Study: Western Australia UBCTD Project That Boosts Oil Production
This blog describes a Directional Coiled Tubing Drilling job that was performed in Western Australia. A multi-lateral well was drilled in underbalanced conditions with the aim of increasing the oil production and avoiding formation damage. Placing the wellbore in the correct geological position within the reservoir in order to maximise production was the main challenge. The well under consideration was planned to be drilled horizontally with the objective of providing oil production from the central fault block within the field while intersecting good quality, laterally connected reservoir. The underbalanced drilling was aimed to maximise production rates at low draw down pressures through a greater pay interval and to minimise formation damage. The horizontal section was designed to maximise lateral drainage of the reservoir as maximum length was limited by the anticline and overlying shale. Therefore, the placement of the wellbore had to be as accurate as possible in order to deliver the longest lateral achievable in the most productive portion of the reservoir. AnTech took advantage of RockSense, a real-time, at-bit, synthetic porosity signature software for bed boundary identification. This technology offers two considerable advantages over traditional geosteering techniques. Firstly, operators can gain inch-level resolution because multiple measurements can be made for every foot drilled, due to the fact that wired telemetry has a high data rate. Secondly, formation boundaries can be detected at the bit, not 20-30 ft behind it, as the software can tell the directional driller whether a bed boundary has been passed, within inches of the BHA passing through it. Therefore, the driller can deliver an optimally placed wellbore and respond to changes in formation as the bit passes through them. In this particular instance, the reservoir was a homogeneous dolomite with very little gamma character. Therefore, RockSense provided valuable insight into the formations vugular nature, fractures and faults. The sidetrack was landed within the reservoir’s crest structure at an inclination of 89.5 deg, as tight as technically possible after leaving the casing point, keeping the wellbore as structurally high as possible. Total Depth (TD) was called when gamma and RockSense readings were showing the bit was approaching the overlying shales. The well achieved very encouraging initial production. This demonstrates the benefits of RockSense and the impact of underbalanced CTD when combined with expertise in both surface and subsurface operations. Author: Matteo Gemignani
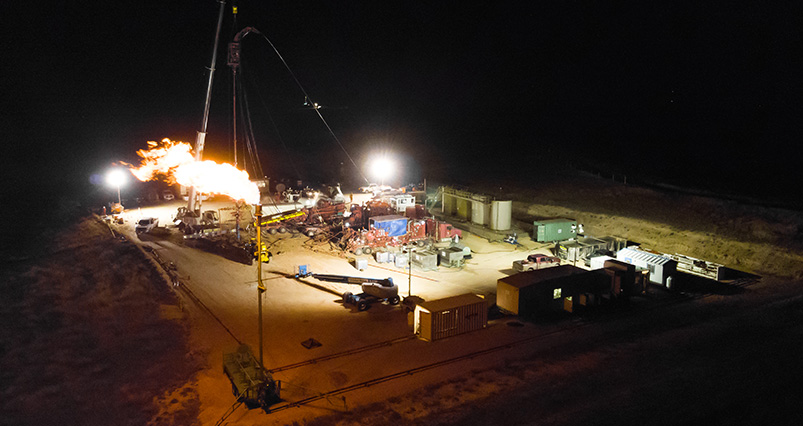
1588334400000
Case Study: UBCTD That Enables Real-Time Decision Making
This blog focuses on the capabilities of Underbalanced Coiled Tubing Drilling (UBCTD) demonstrated in a project led by AnTech in the Texas Panhandle. The project was a two well re-entry campaign, aimed to intercept fractures and access untapped reserves to boost production. Underbalanced drilling was performed to prevent overbalanced conditions that can have a significant impact on production. A stable underbalanced system allows reservoir characterization while drilling and it is especially important when working with a two-phase fluid. The wells under consideration were gas producers with condensate and water also being produced. The areas of particular concern were the stability of the build section in the overburden above the pay zone and the risk of hitting water-bearing fractures in the horizontal section within the reservoir. For these reasons, a great deal of time and effort was given to planning the operation in order to drill the wells as planned to avoid any surprises and mitigating challenges. An expandable liner was kept on standby as a contingency for the wellbore stability and inflatable plugs were made available in case of hitting waters. The parties involved in the project were: the Directional Drilling company (AnTech),the Coiled Tubing service provider (Halliburton),the flow back provider (ADA)and the Operator (Winright Resources) The role of aggregating the data from all parties involved was also taken by AnTech, by deploying digital technologies in the form of wellsite data acquisition and office support for data analysis and downhole sensors. This allowed all parties to have a complete picture of what was happening in real-time on the wellsite and the speed of decision making could thus be improved. A workover unit was used to pull the tubing out and carry out the casing exit operation in both wells. The first well was then entirely side-tracked with Coiled Tubing Drilling. The build section of the second well was instead drilled and cased conventionally due to wellbore instability caused by shale intervals; the horizontal lateral was then drilled with Coiled Tubing. This project is a good example of the importance of thinking of an UBCTD campaign as a whole, in which the Return on Investment (ROI) was maximised by using the technologies where they were best suited. AnTech’s gyro technology was used to pass through the window in the first well. It provided Gyro While Drilling (GWD) capabilities and was used to take a survey and orient the bent housing motor to pass through the window. The technology is incorporated in the BHA in order to assist with passing through windows without getting stuck and for dealing with inaccurate magnetic surveys when close to the existing casing. Productive fractures and faults were identified by utilising RockSense in combination with production data. The well proved productive until a water bearing fracture was intercepted. It was decided to stop drilling and run two inflatable plugs to seal off the water bearing fracture. RockSense identified the exact location of the fracture in order to set the plugs as close as possible. The project showed that UBCTD, combined with aggregation of digital surface data, can be run successfully in the challenging US land environment. It also validated the technology and provided a proof of concept to be taken forward to the Operator’s future projects. The combination of technologies deployed gave four key benefits to the operation. It enabled the wells to be drilled with zero formation damage. It enabled the use of RockSense which meant real-time information on fractures. It enabled real-time data aggregation and therefore real-time decision making. It enabled live well testing and therefore removed the delays inherent in gathering data and analysing it off site before proceeding. Author: Matteo Gemignani
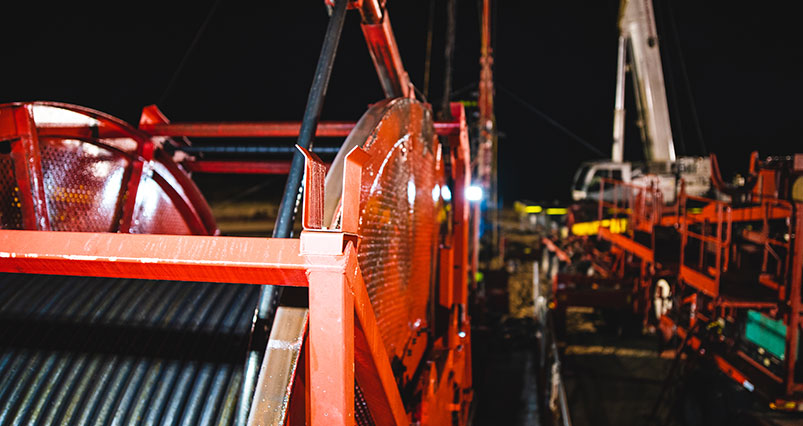
1579176000000
Benefits Of Underbalanced Coiled Tubing Drilling
Underbalanced drilling (UBD) is an important technique for drilling mature fields with pressure-depleted reservoirs, narrow pressure windows and/or drilling issues. It can be illustrated as follows: The bottomhole circulation pressure is a combination of hydrostatic pressure, circulation friction pressure, and surface pressure applied at the choke. When drilling underbalanced, the well is still controlled by maintaining the wellbore pressure, but this pressure is kept to always be below the reservoir pressure. In underbalanced drilling, the primary well control function of the mud column is replaced by a combination of flow and pressure control, while secondary well control functionality is provided by the BOP’s. UBD increases reservoir productivity and maximizes net present value by minimizing or avoiding near-wellbore damage and enhancing reservoir characterization. To design and manage drilling operations on an underbalanced well, as with any drilling project, significant pre-planning is required for successful implementation of the job. A better ROI is one of the main reasons to drill a well on coiled tubing, in underbalance conditions. Either the cost of drilling the well must be lower or the well productivity must increase once the well is drilled. CTD design and manage drilling operations on an underbalanced well, as with any drilling project, significant pre-planning is required for successful implementation of the job. A better ROI is one of the main reasons to drill a well on coiled tubing, in underbalance conditions. Either the cost of drilling the well must be lower or the well productivity must increase once the well is drilled. Using underbalanced drilling with coiled tubing can benefit a project in three ways: Significantly minimises pressure related drilling problemsReduces formation damage and increases productionReservoir evaluation while drilling Major benefits of underbalanced drilling over conventional overbalance techniques can be summarized as follows: Reduced formation damage leads to increased well productivityReduction in lost circulation of drilling fluids into the formationEliminates differential stickingIncreased ROPReduced completion costsDiminished or absent stimulation requirements and costsImproved formation characterisationExtended drill bit life Appropriate candidate wells need to be screened for applicability of UBCTD. The key to selecting the right candidate is reaching a balance of technical, safety and economic factors. Author: Marat Seitimov
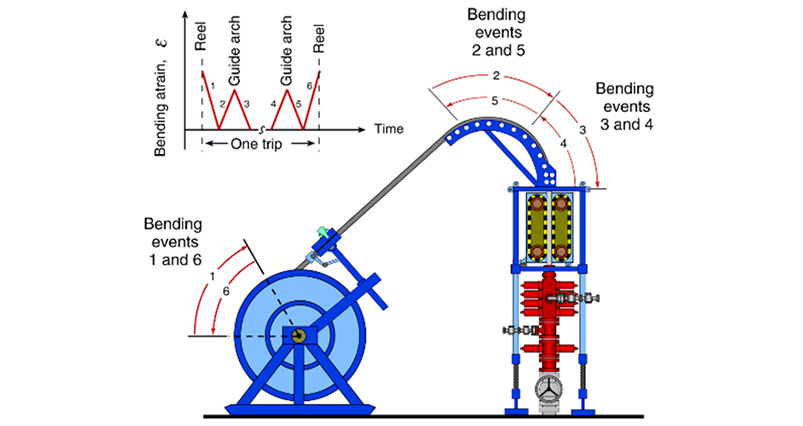
1566388800000
Coiled Tubing String Retirement
Fatigue life modeling has extended the economic life of Coiled Tubing (CT), making the ability to predict the safe working life of CT string is an essential part of coiled tubing operations. New applications like drilling and fracturing can now be undertaken with confidence and success, revolutionizing the CT industry. A coiled tubing string becomes unsuitable for continued use if the accumulated fatigue exceeds the limits set by the operating company. There are several methods utilized to predict the service life of the coiled tubing string. Some are quite simple; some can be more complex. The simplest approach that is easy to implement would be a “running-feet method”. A total footage for the CT string run into wells is recorded, when a specified limit is reached, a decision to retire the string is made. Typical length would depend on the CT string properties, and can vary from 250,000 ft up to 750,000 ft. With new coiled tubing developments, this range limit can now be increased. However, the range limit is based on the previous experience with similar tubing performing similar operations under similar well conditions. A company cannot simply extend the same limits to a different size string or different well operating conditions as the fatigue life will be different. An upgrade to the running-feet method would be to account for variations in the stresses along the string. Typically, certain sections of coiled tubing string are subjected to more frequent bending over the reel and gooseneck, therefore, reducing the fatigue life of those sections faster comparing to the rest of the string. Recording accumulated fatigue in each section, typically 500 ft long, will help to get more accurate results of the string condition. Currently, when a certain section or sections of the tubing reach a fatigue life limit that was previously set by the company, the tubing is cut from the bottom, so a highly fatigued section would be positioned deeper downhole during subsequent operations. In turn, a section with higher fatigue life would be on the reel and gooseneck and operate until it reaches its limit as well. The CT engineer must be aware of the well depth that the string is going to be used in, to make sure that the section with the least fatigue life is not subjected to high bending stresses during operation. The length of the tubing removed should be at least the distance between the reel and the guide arch. If necessary, additional pipe section might be cut-off to adjust for string placement in the well. If the CT string still has enough fatigue life left, but has reached a certain length, where it is no longer suitable to operate in the given well depths in the region it operates, it is time to retire it or it can be re-sold to a region where existing wells are shallow enough for that length of tubing. Loss of the integrity of any tubing string in any condition makes it unfit for the service. Fig. 1 - CT String Accumulated Fatigue Lastly, the most accurate model to predict fatigue life of the string is theoretical modeling. It involves using various algorithms to predict and estimate the fatigue damage due to proposed CT operation. String Management software allows tracking the fatigue status of a CT string in order to avoid costly failures at the wellsite while maximizing the usage of the pipe before it is retired from Service (Fig. 1). The essence of fatigue tracking is to know exactly which section of pipe is being bent at any given moment, and what the prevailing conditions are at the time. Author: Marat Seitimov
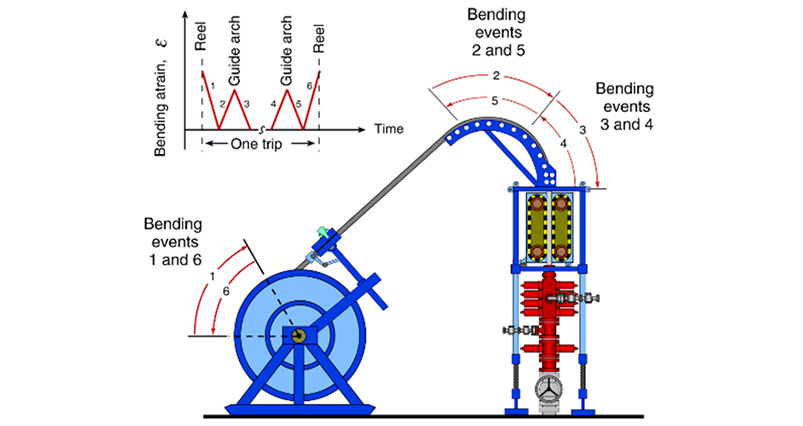
1563364800000
Coiled Tubing: Repeated Bend Cycling And Microcracks
Fatigue life measurements appear to be a statistical phenomenon, which makes the measurement of the fatigue life of the Coiled Tubing (CT) string to be only an estimation. The fatigue condition of a segment of the tubing with a known bending history cannot be measured accurately with currently existing tools and nondestructively. You can also expect variations in the observed fatigue life for any tubing string, regardless of comparable bending history records.. Repeated bend cycling of CT string results in a reduction in material yield strength and has a terminology name of “cyclic softening”. When subjected to plastic deformation because of bend cycling with internal-pressure loading, the diameter of the CT tends to grow or “balloon”. As the diameter of the tubing grows, and since the cross-sectional area of the tube body is assumed to be constant, then the wall of the tube gets thinner due to the redistribution of the material. The primary factors that can cause diametral growth of the tubing string are tube outside diameter (OD), wall thickness and material properties, but also bend radius, and internal-pressure loading. However, CT growth measurements in the field are usually much less than the results obtained in lab testing. Primarily this is because of the factors such as tubing rotation, which causes the wall thinning to occur more uniformly. One of the most common tubing failures result from microcracks. As the tubing undergoes a constant cycle during CT operations, eventually, it leads to the formation of microcracks in the tubing body and loss of pressure integrity. Detection of cracks can be extremely difficult - one of the reasons is that cracks start to form on the inner side of the tubing and appear to be very small. However, under high internal pressure, the crack may instantaneously propagate along the circumference of the tube, resulting in a major failure – mechanical separation of the tubing. When evaluating the tubing failures, these microcracks are amongst the most common causes of the CT string failure. Coiled tubing string is an expensive consumable, so service companies try to get the most out of their investment. Maximizing the usage of the string must be in conjunction with minimizing fatigue failures. Most of the coiled tubing companies have their own fatigue life tolerances or limits upon which they decide to retire or cut the tubing. In general, the decision to retire the coiled tubing must consider current tube conditions, its service history, and the anticipated service loading. CT life management software constantly tracks the accumulated fatigue in each section of the CT string. A single job may use up to 80% of the CT fatigue life, therefore, the importance of keeping the string data up-to-date is crucial. What started as a drilling operation may end as a fishing job and may cost the operator significant amounts of time and money and may hurt the reputation of the service provider. Author: Marat Seitimov
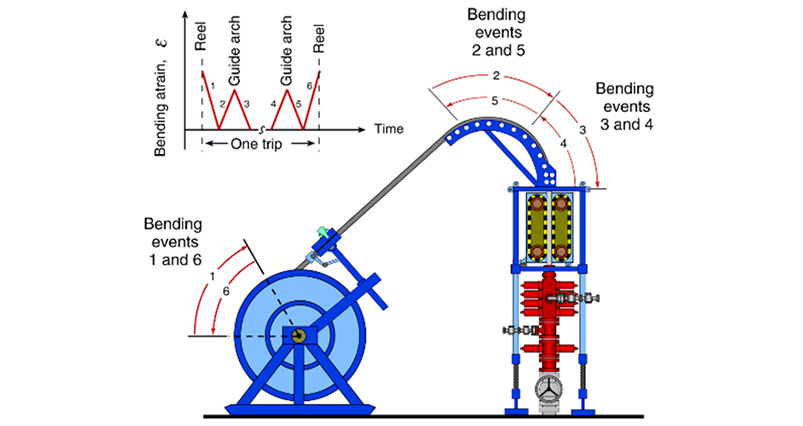
1560340800000
Understanding Coiled Tubing Fatigue Damage And Its Influencing Factors
Coiled Tubing (CT) has been widely used for well intervention, servicing and drilling. It has become widely used due to its low cost, fast mobilization, and high efficiency. The CT unit’s major consumable is coiled-tubing pipe, which is rolled onto the reel (drum). The tubing moves from the reel over the tubing-guide arch, called a gooseneck, repeatedly during operation and is usually under constant internal pressure loading. This leads to the fatigue damage of the tube; therefore, it is very important to keep track of the most fatigued zones to prevent any pipe fractures during operations that could result in tubing and BHA loss. We must note that the fatigue factor in the life of CT is unavoidable, and currently cannot be measured non-destructively. Foremost, to reduce the likelihood of coiled tubing string failures and improve reliability of the operations, it is critical to understand the mechanisms of the CT fatigue damage occurrence, and how to predict string fatigue condition. There are three major classes that identify fatigue. They are: High-cycle fatigue, low-cycle fatigue, and ultra-low cycle fatigue. Coiled tubing fatigue falls into the last category ULCF. Another example is a paper clip that fails after a few bending cycles. As seen from Fig. 1, there are a total of three bending and straightening events, which are repeated in reverse as the tubing is going down the well or out of the well (in the oilfield it is commonly described as a “trip”), resulting in a total of six bending events. Research has found that this segment of the tubing will have a higher amount of fatigue damage accumulation. Failure of the CT caused by repeated bending on the reel and over the gooseneck has been a major concern for coiled tubing service companies and operators that use their services. It should be noted that the number of trips that coiled tubing string experiences over its entire length is not homogeneous. During routine CT well-intervention and drilling operations, the tubing string is periodically stopped and reversed in motion, therefore, certain segments of the pipe experience a higher number of trips allocated compared to the remainder of the coiled tubing string. The majority of metal fatigue occurs on the reel and at the the gooseneck prior to going into the well. Image Source: For many years there have been multiple studies made to measure fatigue damage using different models. A study made by Newman and Newburn, found that decreasing the coiled tubing (CT) diameter or increasing the bending radius leads to an increase of the CT life.* Another study found that increasing the wall thickness and yield strength will lead to the increase of the CT life. There were other experiments and models that measured different factors on coiled tubing life. Most of those studies are too complex to describe here. Major parameters that affect coiled tubing fatigue life are CT outside diameter, wall thickness, bending radius, operating conditions, and tubing material specifications and properties. There are other factors that should be included in the analysis of the CT fatigue damage, such as tubing ovality, pre-bending, corrosion, mechanical damage, severe operating conditions of coiled tubing inside a well, and rotation. However, these are secondary factors compared with those described above. *Conference Paper DOI: 10.1115/IMECE2016-65972. Author: Marat Seitimov
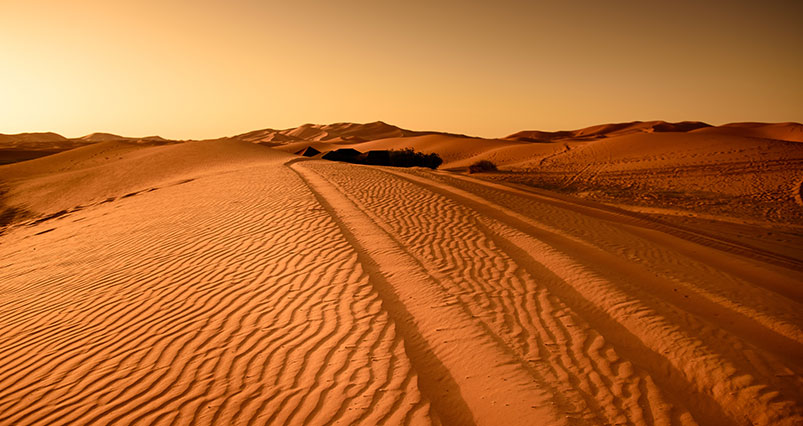
1548244800000
Underbalanced Coiled Tubing Drilling in Tight Sandstone Case Study: Part Two
In a recent blog post we looked at how Underbalanced Coiled Tubing Drilling (UBCTD) has the potential to offer a more than viable method of extraction from tight sandstone formations. To recap, the results of the project we presented in the post were impressive. The production recorded on the preliminary post well flow test showed a production of three times the target flow. (A summary of this UBCTD project can be found here.) What is particularly interesting to note is that the project did not run smoothly and resulted in unexpected additional costs. But despite these challenges and costs, UBCTD still remained an impressively viable option. Overall the project faced excessive tripping time. It took over 30 runs (including two whipstocks and four milling runs) to drill approximately 2,400 ft. Achieving the run in hole was difficult because it involved drilling through an unstable clay-stone formation. However, the accuracy enabled by the UBCTD approach meant drilling could be controlled successfully and enough reservoir contact could be achieved. The second whipstock needed to be installed to reduce the dog leg severity and make it easier to trip in. Again, the accuracy of the UBCTD approach enabled the second whipstock to be situated next to the first. The project saw three laterals being drilled. All three had their total depth called earlier than planned because of drilling difficulties. An impressive flow rate was still achieved in spite of this. The high stage separator saw excessive foam, meaning antifoam had to be injected upstream at the 5k choke. At first glance, it would be easy to assume that drilling using UBCTD in tight sandstone was only economically viable in this instance because the project ran unusually smoothly. But as these insights show, the project was far from seamless. It illustrates that UBCTD is a method that delivers in the real world. Author: Guest Blog by Naceredine Fares
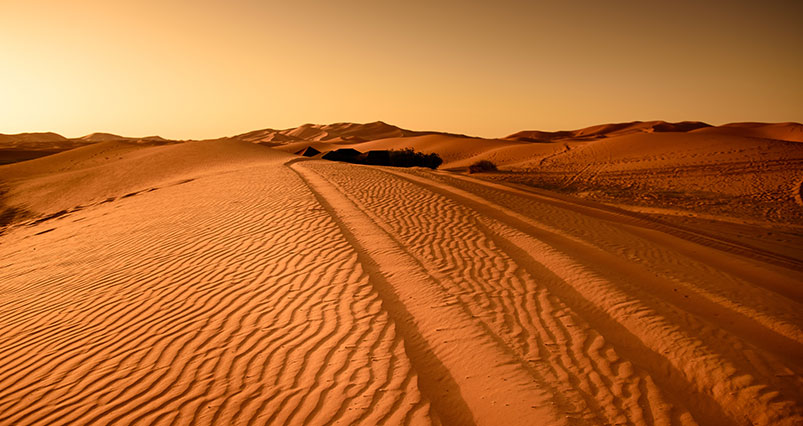
1547035200000
Underbalanced Coiled Tubing Drilling in Tight Sandstone Case Study: Part One
The low permeability of tight sandstone means it is notoriously difficult to achieve a productive well economically. However, it has been demonstrated that Underbalanced Coiled Tubing Drilling (UBCTD) can provide an economically viable solution. Let’s take the example of a tight sandstone field in the Middle East. The logs showed good gas levels that were worth exploiting. The first attempt to extract was done conventionally, using overbalanced drilling. However, this led to production that was next to nothing. It also resulted in damage to the rock around the wellbore. The second method tried was fracking. Again, this failed, even when all the fracking units were maximised. The third attempt involved UBCTD. The results were impressive. The production recorded on the preliminary post well flow test showed a production of nearly three times the target flow. Figure 1 - Wellbore Schematic Here is how it was achieved: A first whipstock was set in the mother wellbore’s tubing. A 10 ft window was milled and a rat hole was drilled to 14,500 ft MD. The first lateral was drilled to 15,020 ft MD. A second whipstock was set in the motherbore because the first was showing an excessive dog leg severity that was having a negative impact on tripping time. A second 10 ft MD window was milled. A further two laterals were drilled. A total footage of 2,360 ft was drilled, with three differentlength laterals. The drilling fluid was caustic water, with use of friction reducer additions to a maximum of 1% to achieve better weight transfer to the bit as the depth increased. Clay inhibitor was also pumped at below 0.5% to inhibit shale. This example demonstrates the value UBCTD has to add in formations such as tight sandstone where low permeability makes other methods of extraction uneconomic or unsuccessful. Author: Guest Blog by Naceredine Fares
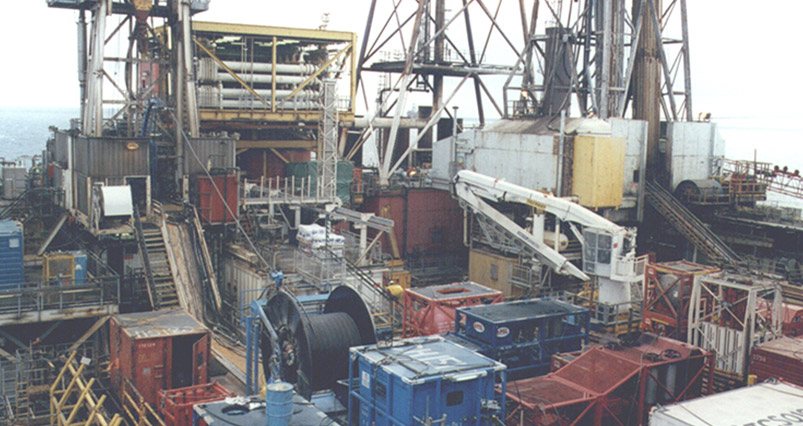
1543406400000
Underbalanced Offshore Coiled Tubing Drilling Case Study: Part Three
With a substantial bulk of the planning for our UBCTD project now under our belts (see parts 1 and 2 of this blog), we’re now in a great position to begin planning the more logistical aspects of the project. Equipment selection With underbalanced drilling there is some additional equipment to be considered and installed. The usual coil equipment such as the coiled tubing reel, power pack, injector head and fluid pumps are all there, but with the well flowing, the return flow needs to be controlled. Therefore, a choke manifold and separation equipment to ensure solids, oil, gas and water all can be separated are also required. In the case of my project, working on a production platform it was possible to have the return flow from the well routed back into the production facilities. Lift gas could be required to achieve an underbalanced status, and this can be sourced from the platform. There is likely a requirement for additional pressure boosters to inject the gas into the coil. The key thing to manage offshore is the solids. Production systems on platforms are not designed to handle drilled solids, so when drilling a sidetrack, special attention needs to be paid to ensure solids are separated out of the flow and shipped back to shore. Space on any platform is limited (as shown in Figure 2 below) and considerable time will be spent on equipment layout design on the deck of the platform. Deck loading, pipe routes, walk ways, access and egress routes and emergency escape routes all need to be considered. Lifting operations need to be carefully managed to ensure loads can be moved without personnel working below. Well control The well control aspects require special attention when drilling underbalanced. The normal fluid barrier is no longer present, and the well is controlled by the stripper rubbers and the choke manifold. During tripping and bit changes, the well needs to remain underbalanced. So once the bit is near surface, a BOP stack needs to be used to ensure the well can be closed and the BHA can be handled. If, for any reason it becomes necessary to kill the well, procedures, fluids and equipment must be in place to ensure this can be done safely. Special attention needs to be paid to flow rates, erosional rates and solids. Equipment can plug or start leaking, so procedures and equipment to be able to control the well become especially important in underbalanced drilling. The image below (Figure 1) shows a gate valve installed below the BOP. This was specially designed so it could be closed when the bit was pulled into the BOP. It saves BOP operations and helps with BHA deployment. Preparation for pre and post mobilization UBCTD requires a lot of equipment to be shipped. As we touched on earlier, when working offshore on platforms, it is important the equipment layout is carefully planned before the job starts. Once the layout plan is complete and all the pipework is routed, it’s important to create an installation sequence. This will ensure that when equipment is placed on the platform, it can still be connected to lines in and out of the well, as well as to the required services of water, electric power and compressed air. Make sure all of the equipment is rigged up in the yard with the offshore crew and ensure pipe work, sensors, cable and walkways can be installed prior to going offshore. Mark out the space you have on the platform and then place and hookup the equipment. This will identify any issues before getting offshore. Piping, walkways and emergency escape routes with the equipment placement and general platform layout are all to be looked at with the entire team, making sure all parties still have access to where they need to go. Conclusion And that’s it, not too daunting right? Joking aside, planning for an underbalanced CTD project can be complex. A successful underbalanced drilling operation requires an understanding of the geology, reservoir production aspects, drilling and finally surface equipment. However, it really is worth the initial hard work, as drilling underbalanced and avoiding reservoir damage means that production rates can significantly improve. Back in the early 2000’s, UBCTD allowed production from the depleted Buchan field in the North Sea to be doubled! Underbalanced coiled tubing drilling onshore converted highly depleted gas fields into successful gas storage fields and allowed additional reserves to be accessed and produced. Yes, underbalanced drilling with coiled tubing is complex, but it is still a lot simpler than doing the same thing with jointed pipe. I hope this blog has been useful to any of you considering, or currently in the planning stages of an UBCTD project. Author: Guest Blog by Steve Nas
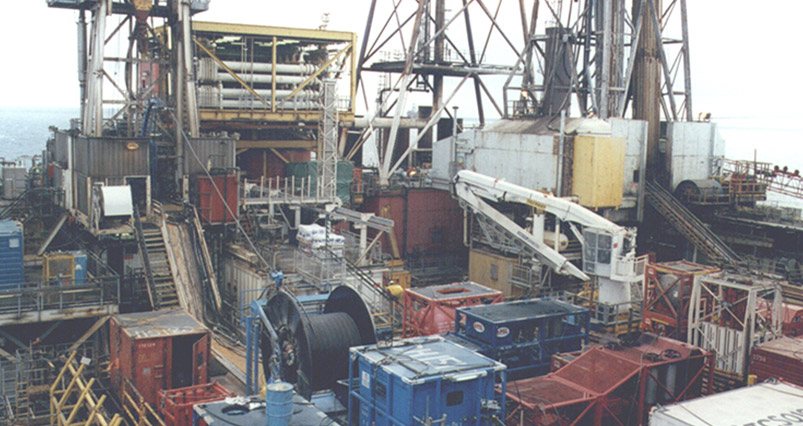
1542801600000
Underbalanced Offshore Coiled Tubing Drilling Case Study: Part Two
In Part 1 of this blog, we looked at the first steps you need to take when planning an offshore UBCTD project, which generally focused on understanding the reservoir. With a preliminary trajectory planned and the well pressures defined, the mechanical aspects of the coiled tubing drilling operation can now be designed. Designing the coiled tubing set-up We now know the hole size and the length of the well to be drilled, therefore we can move on to calculating the coil OD, ID and taper. Using coiled tubing modelling software, the forces at each stage of a coiled tubing operation are calculated and analysed. These include weight-on-bit (WOB), overpull, injector requirements, drag and buckling. We know that friction factors can increase significantly when drilling underbalanced, so ensure friction factors used are well documented at this stage. Do the calculations, then double the friction factors and understand what happens to weights and pulling forces. Look at the buckling of the coil. The OD of the coil is probably fixed but the ID can be adjusted to ensure all the forces work for the well being drilled. The length of coil and its OD and ID will determine the weight of the coiled tubing, and this is an important aspect. The total length of the coil and its OD and ID will determine the weight of the reel. The coiled tubing reel will have to be lifted onto the platform and one of the first questions to be answered is “can the coil reel be lifted onto the platform and where on the platform will it be placed?”, so this needs to be addressed early on. The platform crane needs to lift the reel from the supply vessel and place it where the platform beams can support the weight. BHA requirements With the coil size and wall thickness determined, it is now time to look at the bottom hole assembly (BHA) requirements for the job. An advantage of coiled tubing drilling is that having the wireline installed inside the coil means high-speed bi-directional data transmission is possible. This provides a lot of valuable information that can be used to optimize the drilling process, something that jointed pipe operations can only dream of. Above the bit, two float valves are installed to ensure there are no fluids flowing back up the coil. Above the motor will be an orienter tool which allows the directional control and it allows steering of the BHA. Above the orienter, the data acquisition systems are installed such as PWD, Accelerometers, Gamma Ray, Resistivity, Temperature and any other measurements required. The final piece of the puzzle will be the connection between the bottom hole assembly and the coil itself. Hydraulics and fluid selection Fundamentally, to drill underbalanced, the pressure in the wellbore needs to be lower than the pressure in the formation (reservoir). As we discussed in part 1 of this blog, it is therefore critical that the reservoir pressure is known. A target pressure will be formulated, which is normally approximately 250 psi below the reservoir pressure. This safety margin allows pressure adjustments to be made when drilling. With the reservoir pressure and the required drawdown pressure known, a required fluid density can now be calculated using the TVD of the well. This fluid density will be the first indication of what kind of drilling fluid is required. In a depleted oil reservoir, using the reservoir crude as the drilling fluid is a generally good start. There is plenty of degassed reservoir oil available from the production system and with gas injection, an underbalanced status can generally be obtained. The first objective for the fluid design is to ensure that your well will be underbalanced in a static and circulating mode with a given fluid. This ensures that there will be inflow from the reservoir when the bit reaches it. To ensure an underbalanced status can be achieved, calculations will be completed using multiphase software. The first calculations performed to ensure pressures in the well bore are underbalanced are injection rates vs gas injection and reservoir pressure. The operating window chart provides the operational parameters used to drill the well. A series of six calculations and graphs are generally designed, these are: 1. Bottom hole pressure vs liquid pump rate with gas injection (if required)This calculation shows the pressures when drilling underbalanced and ensures the well is underbalanced when circulating. The graph also shows the amount of gas required to remain underbalanced. 2. Equivalent liquid flow rates through the BHA and bitThis calculation uses both the gas and liquid injection rates to calculate the pressures within the BHA. It calculates an equivalent liquid flow rate and is used to ensure the down hole motor has the required volume to operate, and that the operating window remains within the motor limits. 3. Annular velocityThe annular velocity is plotted to ensure hole cleaning can be achieved. It needs to be looked at in the open hole as well as in potential cased sections of the well. Annular velocities in a 7” or 9-5/8” casing may be too low to bring solids out, especially with coil in the well, so careful analysis of hole cleaning is required. 4. Annular friction pressureThe annular friction pressures are calculated as these play a significant role in UBD operations. The back pressure maintained on the choke manifold controls the bottom hole pressure and the reservoir fluid flow into the well. To ensure the well remains underbalanced, all the factors which determine the bottom hole pressure need to be understood. Bottom hole pressure = hydrostatic pressure + annular friction pressure + applied surface pressure 5. Annular liquid volumeIf both fluid and gas are injected during drilling, it is essential to understand how much liquid and how much gas is in the annulus. If pumping needs to be stopped at any time, the liquid will drop to the bottom of the well, whilst the gas rises to the top of the well. Whether or not the well will remain underbalanced with the pumps off needs to be understood, as it is possible that fluid would need to be removed prior to pumps stopping. 6. Circulating pressure The last of the calculations, this predicts surface injection pressures and is important for selection of pumps and gas compression equipment as well as for fatigue of the coil. The injection pressures in coiled tubing are generally high and both fluid pumps and gas injection equipment need to be sized to ensure required pump pressures are available. Bottom hole pressure versus liquid and gas injection rates With all this analysis completed, we can start looking at the reservoir flow when the well is underbalanced. As we lower the pressure in the well, the reservoir starts to flow oil and gas into the well. This flow adds friction and velocity to the annulus, which influences wellbore pressures. During the planning stage, a lot of scenarios are reviewed to see what happens if there is a gas zone, a water zone and a high producing oil zone in the well, and what the disruptions to the system are. If there is a zone with high permeability in the reservoir, the potential exists to have a high reservoir inflow and this needs to be understood. It may not be possible to drill underbalanced past the high inflow zone because of high fluid rates and associated frictional pressures exceeding the bottom hole pressures. Once operations start, flow modelling will need to be carried out on site to ensure the reservoir inflow performance can be measured. Flow rates of gas, oil and water at surface are measured and correlated to depth to measure the reservoir performance. To be continued . . . Author: Guest Blog by Steve Nas
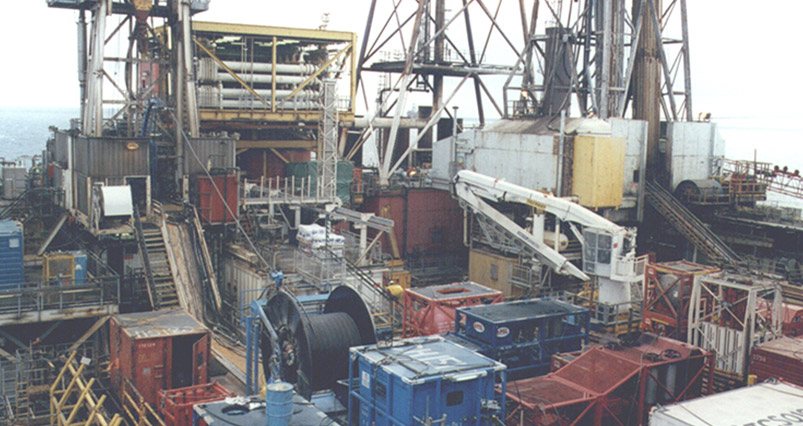
1542196800000
Underbalanced Offshore Coiled Tubing Drilling Case Study: Part One
On a Monday morning, after the operational meetings, my boss walks into our cubicle and drops a pile of paper work, including some old well data, on my desk. He asks me to start working on getting a sidetrack drilled using coiled tubing drilling (the conventional rigs were too busy, so we needed to drill the sidetrack with coiled tubing). As he turns to leave, he tells me the well needs to be drilled underbalanced because the production guys have heard that using underbalanced drilling, significantly increases production. From reading through the well file, I know the donor well is a deviated well on an existing manned production platform. This well is to be sidetracked from a depleted and watered out oil producer and the sidetrack is to be drilled into an undrained section of the reservoir. The target location is partially isolated by faults and is expected to have a higher reservoir pressure. The existing well completion has already been removed and the original perforations are isolated with cement and a bridge plug. So now what? Here, I will outline the key operational considerations that I used when planning this offshore UBCTD campaign. I hope that others will find this useful when planning their own UBCTD projects. Establishing the well objectives The first thing to get right is to establish the well objectives, and understand what the reservoir, geology, geoscience and production teams are expecting. This particular well is an oil producer and the intervention is expected to enhance production from the field. I needed to make sure that all the evaluation requirements such as logging, sampling of fluids, testing and formation samples were clear and well documented. I also needed to obtain detailed descriptions of the proposed new completion and how the completion was to be installed. The completion requirements should provide the first indication of the reservoir fluids and flow rates that the reservoir team have estimated. These factors are critical for underbalanced drilling. At the time, the maximum hole size which could generally be drilled with coiled tubing was up to 6-1/8” hole, but the more usual hole size for offshore drilling is 4-3/4”. Make sure the hole size is reflected in the completion requirements. Understanding the reservoir You need to obtain as much information as you can from the reservoir engineers about the pressures and the fluids. The first things to document are the reservoir pressures and temperatures. It’s important to note here that reported pressure values must always need be verified, as it is critical in underbalanced drilling for these values to be known at the start of the project. The ability to adjust wellbore pressures when drilling underbalanced is limited, and the UBD design needs to ensure the well is indeed underbalanced once it hits the target. If exact pressures are not known, this needs to be reflected in the project risks. Gather all available data on the reservoir fluids, specifically oil gravity, oil viscosity, gas ratios, gas composition and water production. Production engineers should provide answers to issues such as scale formation, emulsions and sand or solids production. The productivity index from the wells (production flow rate versus wellbore pressure and reservoir pressure) provides valuable data for estimating potential flow rates when drilling underbalanced, so make sure you have this information as well as the skin factor currently calculated for the existing wells. Finally, understand where the gas/oil and oil/water contacts are. Drilling a prolific gas cap when you are expecting an oil well can be problematic for a UBD operation. Hitting the water leg can also be a challenge as the system is designed to deal with hydrocarbons and water significantly changes pressures in the well. Define the targets Exact locations and vertical depths are required for all targets of the planned well. Make sure reference depths are related to a known depth datum. Datums can be the Mean Sea Level, swab valve, master valve on the Xmas tree or platform main deck level or even the original rotary table level from when the donor well was first drilled. You are drilling a sidetrack, so ensure depths are all correlated to a single point. As you can imagine, drilling a sidetrack and finding out the reservoir is 120ft deeper than where you are drilling would be a project disaster, so avoid this by ensuring the wellhead and target locations are using the same map geodetic references for the targets. Build your directional trajectory Now that you have your G&G data and targets, it’s time to design a trajectory from the donor well to the new reservoir targets. When drilling with coiled tubing, the possible horizontal departure and reach can be significantly less than when compared to conventional rotary drilling operations. Depending on the depth of the sidetrack and the length of the well, this will be an important part of the design to be reviewed and calculated. Drilling with coiled tubing will allow bigger doglegs, so for a 4-3/4” hole you can accommodate doglegs as high as 5°/100 ft in the well. However, be aware of those completion requirements and associated directional constraints, especially if a completion is to be run after the well has reached TD. Casing/liner requirements With our pressure values and trajectory defined, we can now determine if it is possible to safely drill the sidetrack from the low pressure (depleted) donor well into a possibly higher pressured section of the target reservoir, without isolating the formations. Although pressures can be managed to some extent when drilling underbalanced, once the bit is pulled back into the casing and the well is shut in at surface there should be no cross flow (a nice word for underground blowout) between the various reservoir sections. If an additional liner is required to isolate the lower pressured depleted reservoir section, it is likely that a conventional rig will be required to install it. This does not eliminate coiled tubing drilling, it simply means more pre-work is required using a conventional rig, prior to the coiled tubing drilling operation commencing. Formation stability and pore pressures along the wellbore and possible loss zones all need to be identified. Shale formations can collapse when drilling with low wellbore pressures, and wellbore stability is a key factor for underbalanced well design. Ideally, the casing shoe should be set inside the target reservoir. This casing will isolate any weak shale formations as well as isolating depleted reservoir zones. But in any case, it’s important to be aware that there is always a possibility that weak shales are encountered in the reservoir. To be continued . . . Author: Guest Blog by Steve Nas
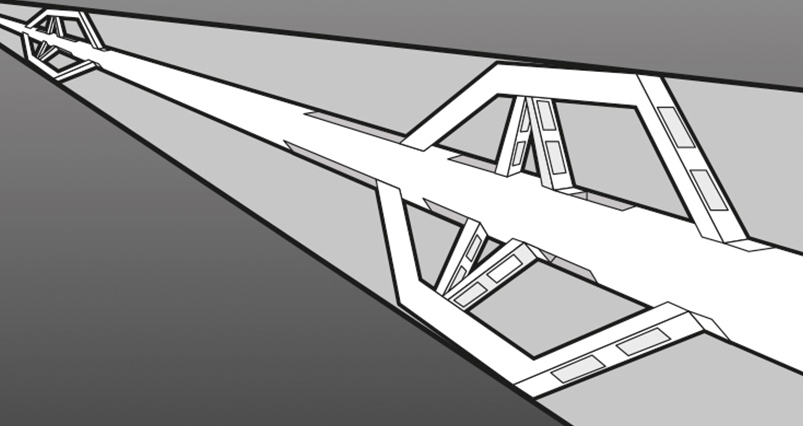
1539172800000
Possible use of Tractors for Coiled Tubing Drilling
Directional Coiled Tubing Drilling (DCTD) is a highly effective and efficient method of accessing tight and isolated reservoirs. The ability to drill extended laterals with precise depth control means that even relatively impermeable formations may be exposed over sufficient length to be economically productive. The ability to side-track existing wellbores and steer accurately to isolated, undrained targets revealed by 21st century seismic techniques breathes new life into old assets. But there are limits. The laws of physics set an upper limit on the horizontal reach of coiled tubing, as we discussed here. One way of overcoming these limits is to use a Tractor. If this conjures up an image of a red machine with black knobbly tyres, think again. A Coiled Tubing Tractor is a BHA component whose job is to engage with the walls of the borehole and push the BHA along, augmenting, and in the extreme, replacing the push provided by the CT. In the realm of CT drilling, we’re typically talking about borehole sizes less than 5 inches. A market leading manufacturer of CT Tractors has products of this diameter capable of providing an additional 9000 lb of Weight On Bit, which can translate in to several thousand extra feet of hole drilled. Of course nothing in this world is free. These extra feet will come at a cost of additional deployment length, and additional valuable metal in the hole. The need for careful planning and pre job simulation becomes even more important. A CT tractor makes it possible to get the BHA so far along a lateral that it becomes impossible to retrieve simply by pulling at surface. Yet in the right place, with the right engineering back up, the coiled tubing tractor, mated to a world class Coiled Tubing Drilling BHA represents a piece of technology whose time has come. Author: Richard Stevens
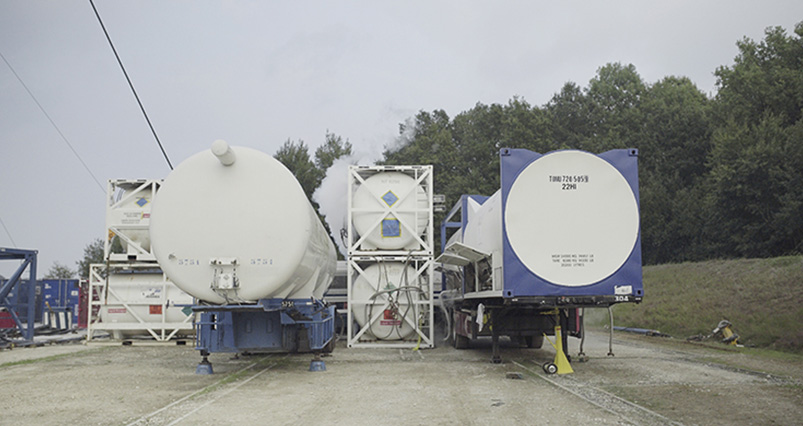
1538136000000
Cryogenic vs Membrane Nitrogen in CT Applications: The Big Picture
For many drilling operations within the upstream oil and gas industry, nitrogen is an essential consumable and is required in significant quantities. In particular, underbalanced drilling operations require nitrogen to be added to the drilling fluid in order to reduce the hydrostatic pressure sufficiently to prevent fluid loss into the formation. The conventional method of supplying nitrogen gas to drilling operations is by utilizing cryogenic technology to transport and store liquefied nitrogen. Nitrogen is transferred from specially insulated vessels into wells using cryogenic pumps, which maintain the low temperatures required. However, the technology is problematic for several reasons. Large quantities of nitrogen are often consumed due to the requirement of high pumping rates, as well as substantial wastage. Not only is it expensive to procure such volumes of nitrogen, but it can often be difficult to source the required quantity in proximity of the wellsite. This results in higher transport and storage costs, as well as the associated environmental impact. Moreover, the use of nitrogen at extremely low temperatures (-196 degrees centigrade) poses safety risks to anyone coming into contact with it during transport or on the wellsite. An alternaitive approach For many years, an alternative method for the provision of nitrogen in certain drilling operations has been to generate nitrogen gas in-situ at wellsites using nitrogen generation units, with ambient air as the raw input material. Within these units, air is compressed, cooled and dried, before being cleaned and heated for carbon absorption and membrane filtration. Activated carbon and particulate filters remove organic contaminants and solid particles down to 1 micron, before the air is further separated by sophisticated membrane filters to produce a ‘clean’ nitrogen gas. In-situ nitrogen generation has several key benefits over conventional cryogenic nitrogen, the most obvious being that one self-contained nitrogen generation unit is all that is required to provide an unlimited supply of nitrogen directly at the wellsite, eliminating the need for costly and logistically challenging transport and storage. In addition, the overall volume of nitrogen required can be reduced due to lower pumping rates, which, in conjunction with the reduced infrastructure required, reduces overall environmental impact. Finally, unlike cryogenic nitrogen, there are no safety implications relating to the handling and transport of in-situ generated nitrogen. However, in-situ nitrogen generation does not produce pure nitrogen gas. In fact, nitrogen gas produced by this process can contain up to 8% residual oxygen components, and this can prove highly problematic for certain downhole applications. Challenges of utilizing in-situ generated nitrogen with CTD Coiled Tubing Drilling (CTD) has long been the desired technology for re-entry operations into depleted wells, thanks to its proven financial, environmental and technical benefits over conventional drilling. As similar such benefits can be found in the use of in-situ generated nitrogen gas, it is common for this technology to be used in coiled tubing operations. The problem arises when coiled tubing comes into prolonged contact with residual oxygen component in nitrogen produced by membrane filtration in-situ, which, under downhole pressure and temperature conditions and with high fluid velocity, can create severe corrosion of the coiled tubing material. Moreover, the effects of this corrosiveness are exacerbated in operations where fluid systems such as completion brines and acids are used. Not only can the coiled tubing be susceptible to corrosion, but also the bottom hole assemblies (BHAs) can stop functioning due to blockages caused by build up of corroded metal from the coiled tubing. Therefore, despite its benefits over cryogenic nitrogen, in theory, the use of in-situ generated nitrogen can be extremely problematic. A reliable solution? When operating membrane units well below their rated capacities they can produce relatively clean nitrogen with low oxygen levels. Unfortunately, this is often impractical and will not meet the nitrogen rates required for the operation. Fortunately, commercial chemical inhibitors are routinely used to reduce the rate of corrosion of the coiled tubing. Early studies indicated that the most successful chemical inhibition strategies included the combined use of oxygen scavengers, phosphate or organophosphonate ester and filming amines. Unfortunately, such studies have been unable to create test conditions that accurately replicate the downhole conditions experienced in underbalanced drilling, and as such these strategies cannot be considered a reliable solution to the problem of corrosion in CTD applications. However, more recent studies that have attempted to create test methodologies using high pressure/temperature conditions have been successful in identifying chemical strategies that provide significant inhibition against the oxygen corrosion of coiled tubing experienced in underbalanced drilling operations. A phosphate ester inhibitor formulation with a controlled near-neutral solution pH and additional bivalent cation was demonstrated by McNealy et al to be capable of producing a stable protective film without the production of corrosion product scales known to cause problems in CTD operations. Therefore, it can be recommended that in-situ generation of nitrogen gas can be an effective solution (and indeed more beneficial than the use of cryogenic nitrogen) in underbalanced applications providing it is either used in a certain way (at lower capacities), or that chemical inhibition formulations against corrosion are carefully selected according to the specific conditions. Author: Toni Miszewski
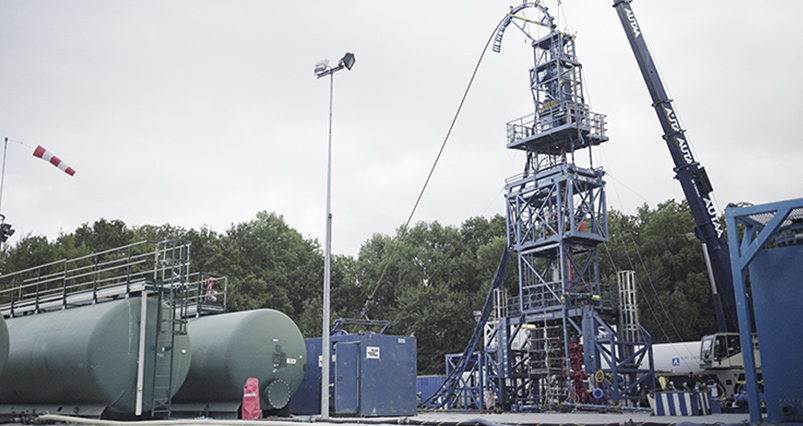
1528891200000
Casing Exits For Coiled Tubing Drilling Operations
Many wells drilled with underbalanced coiled tubing drilling (UBCTD) require a casing exit system to create a window in the original well through which the drilling BHA can pass to drill the sidetrack. Casing exits can be carried out using whipstocks set on coiled tubing, workover units or drilling rigs. Whipstocks are available for both monobore and through tubing applications on coiled tubing. There are two common methods for setting a whipstock on coiled tubing – either as two runs, one for the anchor and one for the whipstock, or in a single run where the whipstock and anchor are run together and oriented using an orienter. When exiting a vertical, or near-vertical wellbore, both systems require a gyro to be used to take an azimuth survey of the whipstock orientation due to the magnetic interference inside the casing. In the case of the single trip system, this means that the gyro must be integrated into the BHA but for the two-trip system a wireline truck could be called off to run the gyro survey on the anchor once set. When evaluating your CTD project you should assess the best method for carrying out casing exits. In some land operations, it makes commercial sense to utilise a workover unit to carry out the casing exit due to low cost and high availability of workover units. In other locations, for example offshore, it is better to carry out all the operations using coiled tubing due to issues with mobilisation and cost. When planning a UBCTD re-entry campaign it is important to decide your casing exit strategy and analyse the best method for realising the campaign objectives within budget. If utilising workover units to carry out the casing exits (and potentially to run completions) then a particular focus should be placed on the scheduling of the workover and CTD operations to ensure the minimum cost and the earliest first oil. If the decision is made to carry out the casing exit on coiled tubing then it is advisable to use a Milling Monitoring Tool (MMT). The MMT gives real-time downhole WOB, TOB, internal and external pressure, and vibration readings. This allows the operator to optimise the milling parameters and therefore minimise the time spent milling the window. Author: Adam Miszewski
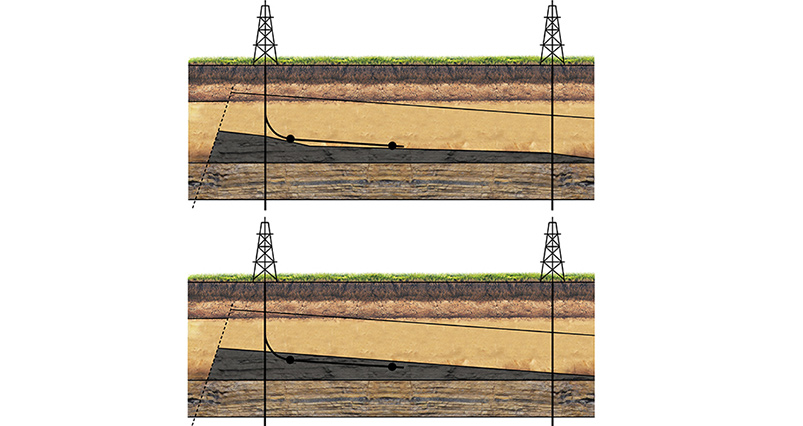
1524052800000
Real-time, at-bit Geosteering for Coiled Tubing Drilling
When drilling a directional well, predicting the exact position of a target reservoir from surface is difficult. Logs from offset wells provide reference points but little indication of the formation away from the wellbore. Seismic measurements lack the resolution to fully complete the picture if the target zone is very thin. This is where geosteering is required. Traditionally, geosteering is performed by analysing the cuttings returning from the bit, or, with LWD logging tools positioned back from the bit. Both of these methods involve a delay between the time that the bit drills a formation and the time that the formation is identified. Depending on the depth of the well and the ROP, this delay could be over an hour. Unlike conventional drilling with jointed pipe, coiled tubing drilling communicates at high speed with the downhole tool over wireline. This is a tremendous comparative advantage and provides near instantaneous feedback of the drilling process. Using the full range of sensors that are available on the latest generation of CTD BHAs, it is possible to process the measurements, while drilling, to determine how hard it is to drill through a particular rock formation. This is similar to the way that, when drilling into a wall with a hand drill, it is possible to get an idea of the material being drilled through. With this capability, it is possible to discriminate one formation from another with a resolution of as small as 6 inches along the length of the wellbore. These changes can then be compared to offset logs to provide a geosteering capability. CTD geosteering technology, such as RockSense, offers considerable advantages over current methods which are accurate but suffer from limitations. The technique of observing cuttings suffers from the time required to pump them to surface, the physical spreading of the cuttings in the well and the time it takes for a geologist to analyse them. Expensive LWD tools in the drill string may be several tens of feet back from the bit so may not pass the zone of interest until an hour or two has passed. CTD geosteering provides near instantaneous information about the conditions at the bit, and fine linear resolution. The directional driller can determine which formation is being drilled by comparing to logs prepared by the geologists. The well trajectory can be adjusted to keep the drill bit in zone thereby avoiding the overshooting that can be caused by other geosteering methods. An overshoot is bad in many ways because not only is the wellbore drilled into unproductive zone, or worse still, a water zone, but the well trajectory then has to be corrected to bring it back on target at which point it might overshoot again. During all this time, drilling costs are being incurred and productive zone is missed. To help visualise the situation, consider how difficult it would be to keep a car on the road in thick fog if there was a one-minute delay in the time the steering wheel was turned and the time that the front wheels responded. Time-delayed geosteering data is an everyday situation in today’s oilfield and CTD geosteering serves to improve the situation. The technology offers a step change in the predictability of coiled tubing drilling because it makes it easier to ensure that a wellbore is drilled in the right place, the first time. Predictability reduces the financial risk to operators and provides an increase in drilling efficiency. Author: Toni Miszewski
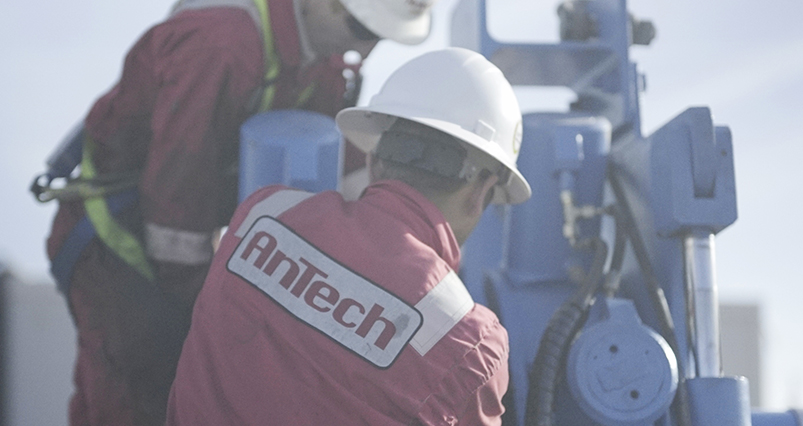
1523448000000
Find Your Reason Why: Well Selection For UBCTD
There are many reasons why you would want to drill using Underbalanced Coiled Tubing Drilling (UBCTD). Not only do you have the advantages of underbalanced drilling, such as maximised well productivity and increased ROP, but you also get the advantages of working with CTD where you get continuous underbalance, fast rig up, small footprint and high build rates. However, even with many advantages, UBCTD has a specific range of applications that it is suitable for. Outside this range, it will be ineffective or completely unsuitable. The key to every UBCTD project is that there is a well-defined reason for using the technology. A focus on the specific value to be gained from the technology keeps the engineering problem constrained within narrow limits so that the return on investment can be maximised. Other secondary benefits will still be captured from UBCTD but they will naturally develop as the planning and execution progress. When assessing your well candidates consider the ways in which UBCTD could revolutionise your well designs and therefore your return on investment. Finally, here are a few example questions to ask yourself during the process (there are many more, of course): Is the reservoir sensitive to formation damage? UBCTD could increase production more than 3x compared to overbalanced drilling.Could high build rates remove a casing string or liner from the well design?Could you save cost by utilising a smaller pad or by using access roads already suitable for a CT unit but not a rig? More to follow in my next blog… Author: Adam Miszewski
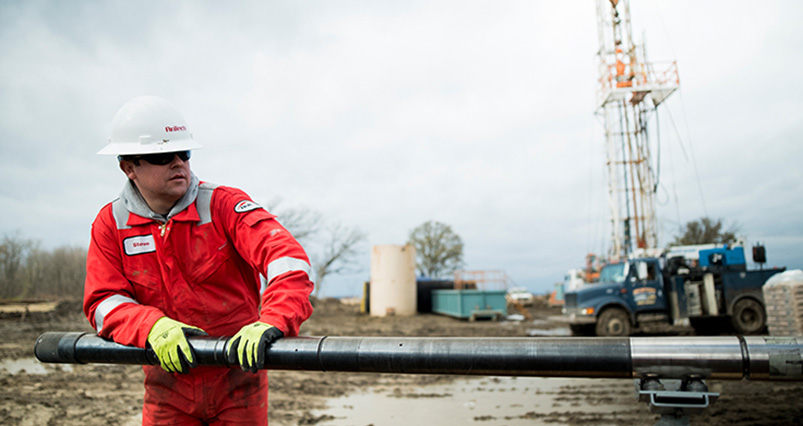
1521374400000
What Is An Orienter?
A directional coiled tubing drilling string comprises a number of highly specialised components working in unison. One of the more interesting components is the orienter. It’s a component whose features we sometimes struggle to explain to clients, so in this article I am going to discuss the purpose of the orienter, its function and give a brief tour of one world class embodiment. To understand why we need an orienter when drilling with CT, let’s consider the case of drilling directionally on drill pipe. To change the direction of a borehole, a directional mud motor (that’s a mud motor with a bend) is conveyed to the bottom of the hole on jointed pipe. An MWD sub immediately above the motor tells us which way the motor is pointing. Clockwise torque is applied at surface until the MWD confirms that the motor is pointing in the direction we want the hole to progress. WOB is then applied and the motor drills away. MWD Surveys are ‘pumped up’ as the hole progresses and any deviation in Toolface can be corrected by modifying the holding torque at surface. Once sufficient angle has been achieved, the same survey package can be used to confirm the azimuth of the new hole. If the measured azimuth is not in accordance with the plan, the drill pipe may be rotated to orient the Toolface to make corrections in the horizontal plane. Now let’s consider the CT case. The directional mud motor, and the survey package above it are conveyed into the hole on CT. CT is continuous pipe, stored on a semi trailer sized reel at surface. CT cannot be rotated in the hole. To do so, the reel, injector and upstream hydraulics would need to rotate around the wellhead in perfect synchronisation. So changing Toolface by rotating at surface is not an option. This is where the orienter comes in. The orienter sits somewhere between the end of the CT and the top of the mud motor. The orienter exerts a torque to enable the drilling motor to rotate relative to the end of the CT, about their mutual axis. It makes sense for the survey package to be located below the orienter, so that the results of an orienting action can be measured directly. Alternatively, a positional transducer on the orienter itself can be used to track the relative positions of the upper and lower BHA and apply appropriate corrections to the survey. If we look at the specs for a typical slim-hole drilling motor, we can see operating torques in the range 400-600 lbf-ft. To do its job, the orienter needs to rotate against this torque so that the direction of the hole can be changed while drilling is in progress. This rating alone tells us that the orienter is no trivial piece of kit. Several implementations have been demonstrated in the field. Direct hydraulic actuation uses hydraulic control line inside the coil to actuate the orienter which operates on a helix/piston principle. Electric over hydraulic actuation uses a downhole hydraulic pump to develop pressure to act on a similar helix/piston arrangement. A limitation of both of the above is that eventually the piston/helix assembly runs out of mechanical travel, limiting the angular travel of the orienter. Another embodiment uses drilling fluid acting on a hydraulic indexing mechanism. Cycling the pumps causes the orienter to index through a pre-determined angle. This embodiment had the advantage of unlimited angular travel. That is to say, a large angular displacement can be achieved by repeated actuations. However, since each actuation requires cycling the pumps for long enough to cause a substantial pressure change at the BHA, it is not particularly suited to applications where a gas phase is circulated, or where tight control of bottom hole circulating pressure is critical. The present state of the art is the direct drive electrically actuated orienter. A permanent magnet brushless DC motor is powered through e-line in the CT. An innovative drive train trades the DC motor’s high speed for torque, producing up to 1000 lbf-ft under stall conditions, to overcome the most aggressive mud motors. The ingenious design manages to leave sufficient space for drilling fluid to flow through the orienter, so operation of the mud motor is unimpaired. The drive train has no mechanical constraints – it can rotate forever, meaning that it is actually possible to drill a straight hole with a bent motor. Because the electric orienter has no effect on flow rates or pressures, circulation can continue unaffected when a steering change is being made, meaning that spurious, differential pressure related torque reactions are eliminated from the steering process. Author: Richard Stevens
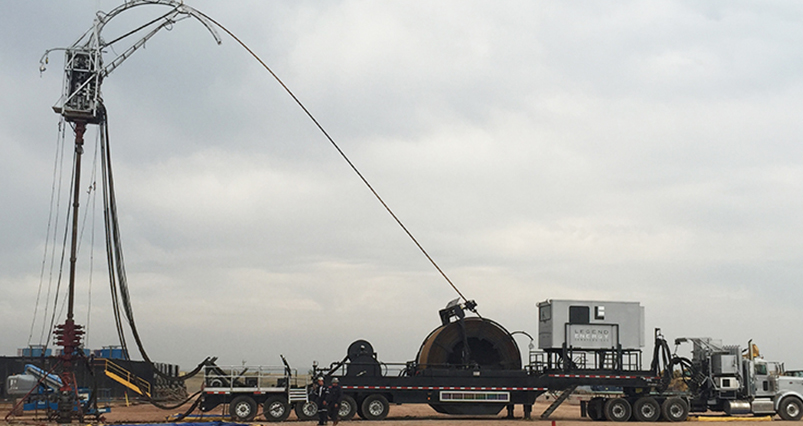
1518091200000
Convert Your CT Unit To E-Coil
Upgrading a coiled tubing unit to e-coil can add valuable versatility to a company’s service offering. The ability to transmit and receive power and/or communications to and from downhole equipment means that a wide variety of smart tools can be conveyed on the coiled tubing including logging tools, perforating assemblies, cameras and MWD assemblies. Technical upgrade of a CT unit to e-coil is relatively straight forward once you have the right equipment to suit your unit. The e-line itself can be injected by the majority of coiled tubing manufacturers upon completion of the string. A number of other companies also own the devices required to inject e-line, including the e-line manufacturers and some coiled tubing service companies. The other components you will need are an electrical slip-ring to allow the coil drum to rotate while not damaging the conductors, a bulkhead for the back side of the coil which will allow the conductors to pass through while containing the pressure inside the coil, and a suitable surface control system to interface with the downhole equipment. When choosing the correct equipment for your CT unit you should take into account the number of conductors required for your application, the maximum voltage and current to be used, any zoning requirements, the maximum pressure and temperature and the design of the drive mechanism for the coil drum. There are two main designs of slip-ring available to fit onto the reel shaft or adaptor plate, and those are flange-mounted and thru-bore. The simplest design to fit to a unit are the flange-mounted slip-rings but some direct-drive drums make these impossible to use because the drive mechanism makes use of, what would be the free end of the shaft, thus making a thru-bore slip-ring necessary. There are also two main designs of bulkhead. Those which terminate the e-line at the bulkhead and use electrical feedthrus to pass the conductors through the pressure barrier, and those which form a pack-off around the e-line so that it can pass through, all the way to the slip-ring. There are advantages to both, in that the former creates a more reliable pressure barrier using o-rings as the sealing components, as well as allowing for easier maintenance and modification on the wire between the coil exit and the slip-ring. The pack-off type has the advantage of being the simpler design meaning that there are fewer terminations that must be made. Once you have all the necessary hardware to convert your unit to e-coil it is very important that adequate training is given to the field engineers that will be running the service. As with all services, the success of the operation depends upon the quality of the personnel as well as the quality of the equipment being used. A good rope socket and conductor termination at either end of the e-line and the correct slack management procedures are of the highest importance to prevent downtime through e-line failure and to maximise the life of the cable. Author: Joe Heaton
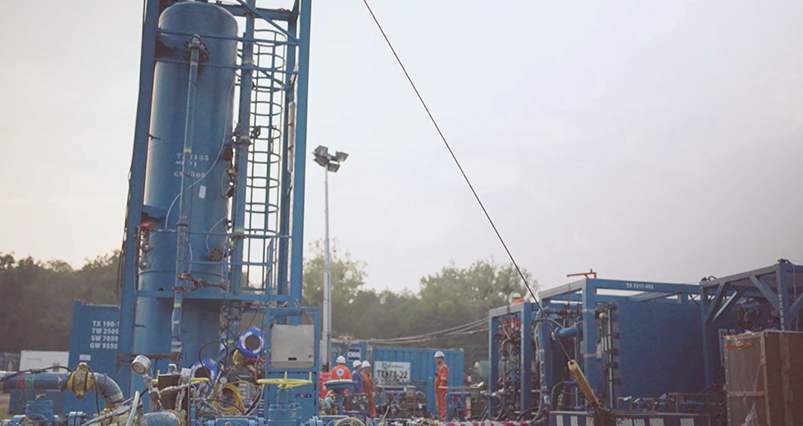
1517486400000
Reservoir Characterisation While Drilling Underbalanced
Drilling underbalanced on coiled tubing provides a treasure trove of information on reservoir characteristics and production. During underbalanced drilling, the bottom hole pressure is kept below the reservoir pore pressure and so the reservoir fluids will move towards and into the wellbore. The response of the reservoir to the underbalanced conditions provides significant insight into its character, including estimates of pore pressure and permeability. At a UBCTD wellsite, data is gathered from the drilling BHA about downhole conditions and the underbalanced drilling equipment, which gives information about surface pressures and production. Typical data collected includes downhole pressure and temperature, production at surface, produced fluid types, among others. This data can be used to characterise the reservoir, while drilling. Characterising and understanding the reservoir is undoubtedly important to optimising a fields development and production. The main benefits of reservoir characterisation while drilling are:· Avoid the time and cost of well testing· Identify zones with production potential that are masked· Improved well completion design· Optimised infill drilling strategy· Improved reservoir management through a better understanding of individual well zones. Coiled tubing drilling is a safe and effective way to drill underbalanced as coiled tubing units are designed and optimised for live well operations. Author: Adam Miszewski
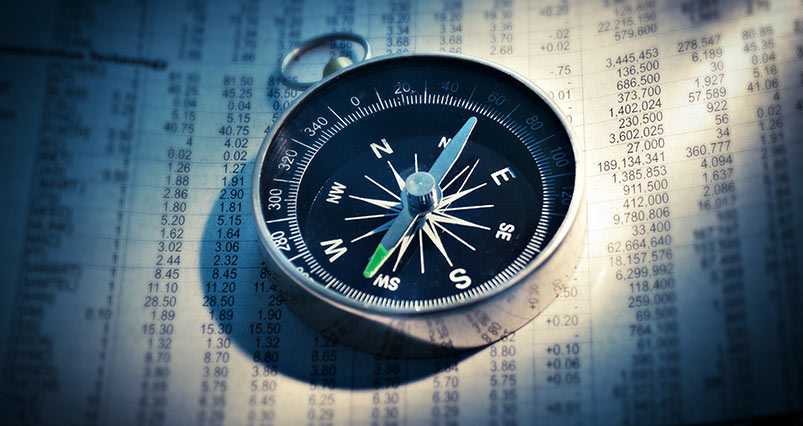
1512561600000
The Importance Of A Gyro In A Coiled Tubing Drilling BHA
If you've spent any time looking into directional measurement instruments, and particularly gyros then you'll know that there are two main types of gyro tool: Integrating (or surface-referenced) and north-seeking. If you've not read too far into it then the key fact to take away is that integrating gyros accumulate errors over time; the longer you run them the less accurate they are. These are commonly used for surveying because here they can be run very quickly and over that time maintain high accuracy. North-Seeking gyros on the other hand measure the spin of the earth itself to get an absolute measurement at each survey point, regardless of the time since the last measurement. These are therefore better suited to measurement-while-drilling applications. The main disadvantage of these is that to gain an accurate reading they need to be perfectly still for several minutes, which usually means shutting off flow to the motor and often this will need to be preceded by wiper trips etc, making the whole process quite a long operation. Running gyros on coiled tubing brings many mutual benefits to these drilling processes. Coiled tubing is great for work-over of existing wells, being able to work in smaller spaces, and with greater ability to control well pressures. Here, the benefits of gyros abound. The surrounding metalwork from the casing and tubing of adjacent wells wreaks havoc with magnetic instruments, and at the same time you've got all the additional safety precautions needed in terms of collision avoidance when drilling amongst live well bores. A high accuracy drilling gyro will provide the confidence required for carrying out these procedures. On top of that you have the ability to reliably run cable down to the drilling BHA for high-speed real-time data. When monitoring the gyro data this gives the user even more control and confidence with the ability to quickly analyse and quality control the survey data and verify that you are heading in the right direction. With the right BHA you can even go from casing exit all the way to horizontal without the need to pull out and change equipment, really unlocking the speed potential of coiled tubing drilling. Author: Benjamin Brooking
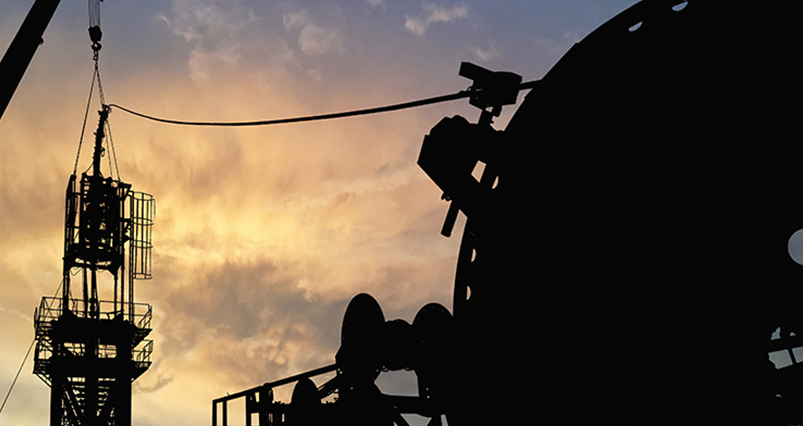
1511956800000
Modelling And Visualisation Of Drilling A Horizontal Well
Let us recall that in academic and engineering contexts, a model is a construct which encapsulates the essential features of a system of interest in a way that offers advantages over direct observation of the behaviour of the system of interest itself. For example, an aircraft designer may construct scale models of a proposed aircraft for study in a wind tunnel. The advantages are numerous: Many different iterations of a model may be manufactured at a tiny fraction of the cost of a full scale prototype, the design of each iteration perhaps being informed by the performance of its predecessor.It is necessary only that a model encapsulates those features under investigation. Therefore, our wind tunnel model maker need only concern himself with shape. Complications like strength, longevity, power and control systems need not concern him, further simplifying the construction of the model.The model may be pushed beyond normal performance limits, to investigate behaviour under conditions of stall, spin, and flutter in a way that would be prohibitively hazardous in a full scale, human piloted prototype.By injecting smoke into the airstream upwind of the model, the flow of air over the shape of the model may be visualised in ways simply not possible on a full scale prototype. To reap these advantages, it is essential that the model accurately represents the behaviour of the system of interest; and a great deal of the effort involved in constructing a representative model is expended in demonstrating that the model provides a true representation of the real world phemomena being investigated. Like generations before him, my eight year old son spends a great deal of time ‘researching’ novel aerodynamic forms based on models constructed from folded sheets of A4 paper. Some of his models are encouragingly performant, but until materials technology has developed to the point where a wing’s thickness may be 1/2000th of its span, his models will not be considered truly representative of shapes that are capable of being manufactured in full scale using state of the art technology. Above we describe a physical model. At the other end of the spectrum, a computational model may be constructed which accurately predicts the resultant forces on a wing of a given geometry in a particular airflow. Nowadays these models are highly sophisticated and robustly validated. A practical result of this sophistication is the commercial flight simulator. Twenty years ago, Airline Transport Pilots routinely honed their skills by switching the autopilot off and hand flying the aircraft during revenue flights. Today’s flight simulators are claimed to provide such high fidelity replication of the piloting experience that pilots are encouraged, and in some airlines mandated, to keep the autopilot on during revenue flights, and prepare for the unlikely event of an autopilot failure by hand flying the simulator during programmed training sessions. We saw above how modelling may enable visualisation of phemomena of interest in ways impossible in a real world system. Let’s consider this in the context of a wellbore. A hole in the ground a few inches in diameter and maybe more than 10,000 ft deep. Imagine it’s a horizontal well, and the end of the wellbore is displaced 3000 ft from us horizontally. It’s a hostile environment down there. Temperatures can exceed 150° C, pressures tens of thousands of PSI, and if we’re drilling, anything down the hole is going to be experiencing percussive forces that can split rock. It’s actually very difficult to observe directly what is going on at the bottom of the hole. Using sufficiently robust electronic sensors we may make measurements of temperature and pressure. With increasing sophistication we can add survey measurements, forces and vibration. Here’s an example of 30 second’s worth of downhole measurements made by a CTD BHA. Doubtless very interesting, and a considerable technical achievement, yet to a human reader, hugely impenetrable. What we really need is a model to help us understand what it means. Here is a 3D graphical model constructed by using a minimum curvature function to join the survey measurements logged as the BHA was pulled out of the well. Figure 1. 3D Graphical Model Unquestionably, using a graphical 3D model makes the data immeasurably easier to understand. We are at a fortunate time in history. Digital computers become more powerful, yet cheaper with each passing year. Computations which could only be dreamed of 20 years ago are now a reality on run of the mill desktop computers. We are approaching the point where our wellbore model will encapsulate not only geometry, tubing forces and hydraulics, but also the mechanical interaction between the BHA, bit and formation, borehole stability and reservoir productivity. In a direct parallel of the flight simulator, it will be possible to drill the well, virtually, in the office, encounter and resolve problems, optimise steering and wellbore placement and simulate hydrocarbon recovery, all before any equipment leaves the yard. Author: Richard Stevens
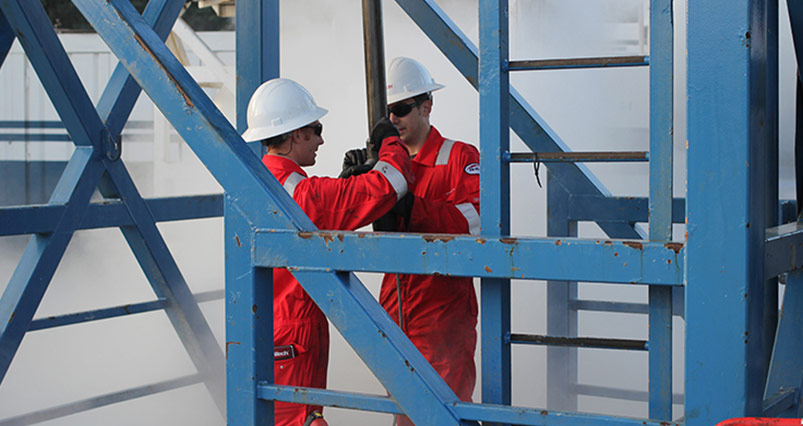
1509624000000
Exploring The Mechanism Of Coiled Tubing Lock-Up
Lock-up in CT is like death and taxes, it’s an inevitability of life. If you are going to try and set weight at the end of CT, the amount of weight you will be able to set will ultimately be governed by lock-up. The key to running a successful operation is understanding how and why lock-up occurs, comprehending factors that influence it and planning the operation to minimise the effect of those factors. These are the topics I Intend to cover in this series of blog articles. Holding a length of coiled tubing in your hand is an instructive experience. In terms of size, weight and stiffness, it’s comparable with scaffold tubes used in the construction industry. It’s readily apparent that a six-foot length, held vertically and balanced on your toe, will exert a downward force equivalent to the weight of six feet of steel pipe, and equally so that a twelve-foot length will exert twice that force. What is not so intuitive is that once the length of pipe you are holding gets longer, it behaves more like a length of garden hose. Most of us can visualise that if we try to compress a short, 8-inch length of garden hose between our palms, that hose will buckle. We can also see that if we hold a slightly longer length vertical, buckling also occurs. In this latter case, it is not compressive force from our palms which causes buckling, it is gravity acting on the hose’s own weight. The relevance of the above is twofold: The force exerted at the bottom end of a length of coiled tubing results from the coiled tubing’s own weight.It is the CT’s flexibility and the resultant interactions with the wall of the hole that cause lock-up. Any long slender member will buckle if sufficient axial load is applied. Consider a wellbore 7500ft deep, completed with 5-1/2 inch tubing, through which a CTD operation will be conducted. The ratio of diameter to length is 1:17000. On the same length scale, our well is in excess of 5 times more slender than a human hair, so ‘long’ and ‘slender’ are clearly appropriate adjectives in this case. The weight of a typical piece of 2-3/8 inch coiled tubing in air is 4.742 pounds per foot. According to a resource* widely respected in our industry, critical buckling load for a vertical tube is given by: Where E is the material Youngs Modulus, I is the second moment of area of the pipe section W is the weight per unit length. Evaluating for 2-3/8 inch CT shows that buckling is likely for loads in excess of about 200 lbf. Accordingly, we can expect 2-3/8 inch CT in a vertical hole to be prone to buckling at depths over 43 ft, as a result of its own weight. Now is the time to make the important distinction between buckling and lock up. Buckling is the state where the CT deforms under compressive axial load so that the CT repeatedly, regularly touches the walls of the borehole, as illustrated in Figure 1. In Figure 1, at every position where the buckled CT contacts the wall of the hole, a component of the axial load carried by the CT acts towards the wall of the borehole. The size of this wall component depends on the contact angle, and hence on the difference between the OD of the CT and the ID of the hole it is passing through. The wall component, multiplied by a friction coefficient, results in an axial force which opposes the motion of the CT. The condition where the sum of these forces exceeds the own weight force propelling the CT down the hole is lock-up. In planning any CT intervention, it is important to evaluate whether the CT and BHA can be conveyed to the end of the hole and set down with sufficient force to operate without encountering lock-up. In this article we have only considered vertical holes. Next time we will look at what happens when we convey CT into a deviated or horizontal well. *CTES Coiled Tubing Manual, 2005. Author: Richard Stevens
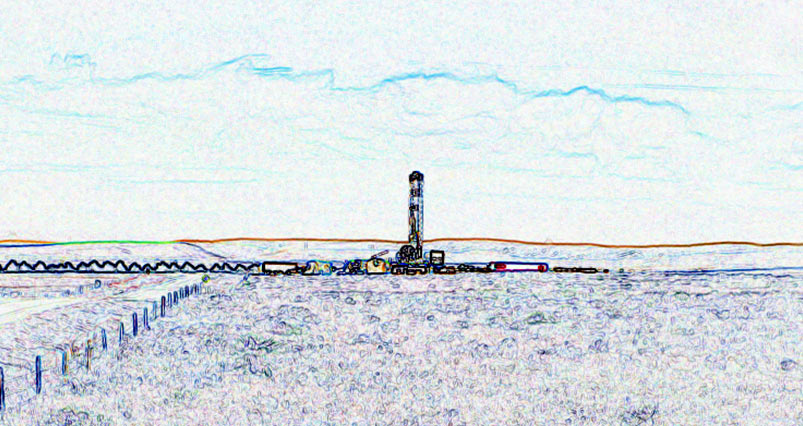
1508328000000
Applications For Coiled Tubing Drilling
With over 70% of the world’s oil and gas production being produced from mature fields, it’s not surprising that operators are looking at ways in which they can increase a well or field’s recovery beyond its decline natural curve. It’s becoming more and more of a focus each year. There are many ways in which an operator can improve recovery such as artificial lift, drilling and completing infill wells or gas or chemical injection but for this blog, we are going to focus on the applications for Coiled Tubing Drilling. The primary reason to use Coiled Tubing Drilling is its ability to re-enter depleted wells quickly and cost-effectively but there are some parallel reasons that sometimes get overlooked. Underbalanced drilling (UBD) True underbalanced drilling or even managed pressure drilling is only possible with Coiled Tubing Drilling. Unlike conventional drilling, there is no need to make connections and therefore breaking the pressure equilibrium or underbalance conditions, Coiled Tubing offers a closed system to control the pressure downhole creating the perfect conditions to achieve at balance or underbalance. Underbalanced drilling also allows the well to produce while drilling, making the payback period much shorter for an operator. Thru-tubing It may not be possible to pull the completion before re-entering the well. Coiled Tubing Drilling is capable of being run through tubing and exiting a completion with a whipstock or a cement plug. The former is generally costlier but often more reliable than a cement plug and therefore the costs may be recouped quickly in efficiency of operation. Lost circulation zones Lost circulation is usually one of the main reasons for an operator wanting to perform managed pressure drilling (MPD) or underbalanced drilling and given that CTD is the only way in which you are able to truly create these types of downhole conditions, it is a great technology for this type of problem. Whether you use MPD or UBD will depend on what you expect the reservoir to produce while you are drilling. MPD may be more appropriate if the equipment is not able to cope with large volumes but the great thing with CTD is that you are able to make adjustments on the fly if you aren’t able to predict this ahead of time. Unconventional gas shales Tight shales do not produce naturally and the ‘go-to’ method of extraction is often fracturing. However, there have been many cases where the need to fracture these tight shales has been completely removed if underbalanced drilling is performed instead, reducing the cost per well substantially. By decreasing the pressure in the wellbore, it allows the gas to flow from the surrounding fractures more easily and avoids any skin damage that may be caused by drilling in overbalanced conditions where the pressure is forcing drilling fluids, fines, clays or cuttings into the rock matrix resulting in the oil and gas flow being impeded. Accessing bypassed reserves and multi-laterals It used to only be conceivable to produce from vertical wells, but in 2015, according to the EIA 77% of wells producing more than 400bopd and 42% of wells producing between 15 and 400bopd were drilled horizontally. Directional Coiled Tubing Drilling not only allows you to drill horizontally, it also allows you to drill many laterals all accessing different zones of the reservoir that were previously bypassed when the well was drilled vertically or even horizontally. High pressure zones Because Coiled Tubing Drilling is an enclosed pressure system that can be controlled, if you were to drill through a highly pressurised zone, expectedly or unexpectedly, any change in downhole pressure conditions would be safely controlled by adjusting the choke at surface and circulating out. Additionally, with e-line running down the centre of the CT, any changes sensed by the CTD BHA will be immediately reported back to surface giving you warning of the changes. These are two of the advantages over conventional drilling, making the operation much safer for people on the rig floor. Coal bed methane extraction (CBM extraction) CBM extraction is a method used for extracting methane gas from a coal deposit. The methane can be extracted by drilling wells into the coal seam. With Coiled Tubing Drilling, the bottom hole circulating pressure can be controlled from surface allowing you to decrease the pressure in the well bore and can avoid the risk of fracturing the coal seam at the same time. The objective is to decrease the water pressure by pumping water from the well and the decrease in pressure allows the methane to be unloaded from the coal and flow as a gas up the well to surface. Underground coal gasification or in seam gasification UCG is the conversion of coal into synthetic gas (Syngas) using controlled combustion in situ underground. By using directional CTD, the combustion process can be controlled much more precisely, making the process much more cost-effective and safer. While CTD may not be suitable for every drilling situation, is it a very attractive application for the above scenarios. Author: Clare Miszewska
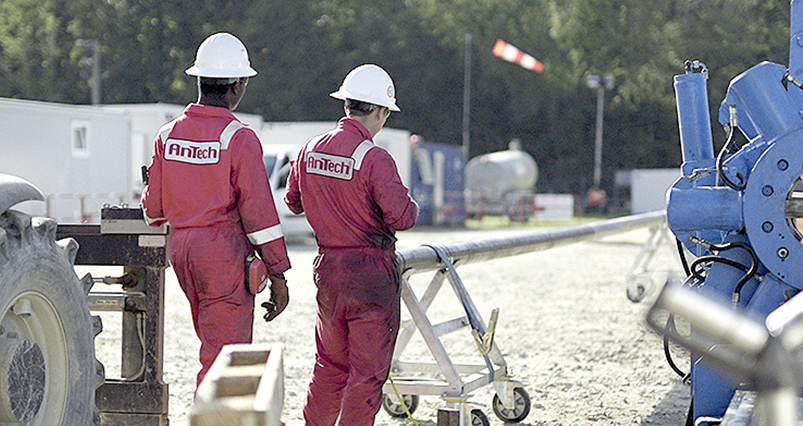
1507723200000
Horizontal Directional Drilling: What's The Benefit?
Since the early days of the oil industry, operators have been drilling vertical wells to tap oil and gas reservoirs. The advantages are cheap and simple wells with minimal need for technology or highly accurate data. Directional wells were limited to situations which required directional drilling to be used like offshore wells or reservoirs with restricted land access at surface, for example. Over the recent years technology has improved to the point where the rewards for drilling a horizontal well have exceeded the risks of failure on nearly all fields. The two main advantages from horizontal wells are: a. more effective drainage of the reservoir (i.e. increased overall reserves recovery) b. reduced water and gas coning. The drainage of a reservoir is increased by horizontal drilling through increasing the amount of reservoir that is exposed to the wellbore. This is also the mechanism behind the reduced water and gas coning, for a given production rate, a horizontal well requires less drawdown pressure and therefore the water and gas coning will happen slower. Directional coiled tubing drilling is usually used in medium radius applications where the build up section can be up to 50°/100ft. This high dogleg capability is useful for avoiding trouble zones when sidetracking from an existing vertical well bore by exiting beneath them. Despite the advantages of horizontal drilling it is still not universally applied. EIA statistics from the USA show that this is changing and the proportion of horizontal wells is increasing. Of the wells drilled in 2015 that went on to produce more than 400 barrels of oil equivalent per day, 77% were drilled horizontally. For wells producing between 15 to 400 barrels of oil equivalent per day, 42% were drilled horizontally and that proportion is increasing rapidly. Only 2% of stripper wells were drilled horizontally. It is clear to see that there is a correlation between drilling horizontal wells and increased production. There are lots of factors at play in such broad statistics but it does show that the technology has come of age and is being used by leading operators to achieve a competitive advantage. Author: Adam Miszewski
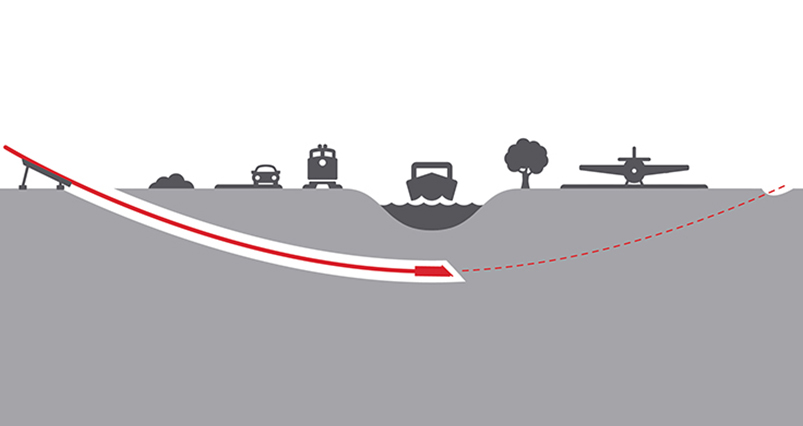
1507118400000
Why A Gyro System Is So Important For Horizontal Directional Drilling
With a background in the oil and gas industry, we’re familiar with the concepts of using different directional measurement instruments to guide us as we carve our boreholes into the depths of the ground beneath our feet. In the 1970s, the nascent directional drilling technologies of the oil and gas industry were taken and adapted to get pipes and cables under rivers River crossing, also known as Horizontal Directional Drilling or HDD was born. Over time, the two have shared ideas, but now as the capabilities of both markets re-converge there’s some interesting advantages to the HDD market in applying some of the latest technology from the oil and gas side. Oil and gas personnel are used to drilling down, and with gravity in their favour, early wells were drilled with no steering and no measurement. Gravitational measurements are all that are needed to validate a perfectly vertical hole, so basic forms of these instruments quickly followed (acid in a bottle, gradually etching marks onto the glass). HDD crews started out life (and start each job, roughly speaking) drilling horizontally. Here again gravitational measurements are key, to control the depth you are drilling at. However, for HDD azimuthal accuracy (your bearing relative to north) is arguably far more important than it is in oil and gas. Even though the horizontal distance travelled is likely to be less, in many environments there are more obstacles to avoid; buried pipe work and cables, house foundations etc. Figure 1. Walk-over equipment used in the HDD Industry Traditional techniques for navigation in the HDD industry include walk-over equipment, which whilst accurate can be impractical where walking over isn’t possible, and magnetic survey instruments which are susceptible to interference. Both are worse in urban environments where the benefits of HDD are great and the accuracy requirements are much stricter. Given all these factors, a gyro measurement instrument becomes the perfect solution, however there is still one hurdle. The most accurate gyro tools for traditional drilling applications are the ones used for logging a hole after drilling. For HDD, it’s too late by then - the driller needs the information as they are drilling. In the oil and gas industry there’s an increasing number of acrobat gyro-while-drilling (AGWD) tools available, but they struggle with the accuracy in horizontal boreholes. An AGWD tool capable of maintaining the highest level of accuracy at all inclinations now exists on the market. Meanwhile, thanks to the increasing level of activity in the development of self-driving cars, the gyro sensor market has considerably upped its game in terms of stability and accuracy. Now really seems to be the time for both markets to make use of ground-breaking (pun intended!) technology for highly accurate horizontal drilling. Author: Benjamin Brooking
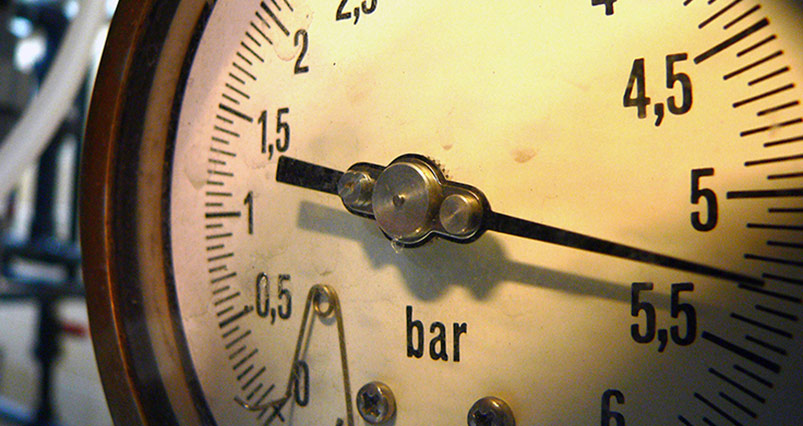
1506513600000
Lesson 101: Setting Up And Operating A Test Facility For HPHT Equipment
Coiled Tubing Drilling is increasing in demand which is creating the need for tools to have higher downhole capabilities. Specifically, the need for higher temperature and pressure capabilities. For this to happen, testing and validation of these tools needs to be performed at High Pressure High Temperature (HPHT) within the workshop before going to the field. There are several challenges in initially setting up the HPHT test facility, these are underpinned by having enough capital investment to set up the facility, cutting corners is not a good thing when dealing with HPHT. The main challenge is having enough space to set up the equipment, tools for CTD are long, (over three metres in length). A typical pressure test vessel for a complete drilling tool could be eight metres long, so having a facility with space to manoeuvre tools and vessels with lifting equipment to do this is paramount. Introducing temperature into the mix with pressure testing, creates a potentially volatile environment requiring high levels of security and safety awareness to be observed and met. Test equipment for HPHT is expensive and the best equipment should be purchased to ensure all safety releases and fail safes work and are adequate for the potential test criteria. For example, your test pressure could be 10,000 psi (689 Bar) and temperature could be 160˚C (320˚F.) The industry is driving this temperature requirement up towards 200˚C (392˚F). Once the facility has been established, personnel training is a high priority, to preserve life as well as your tools and test equipment. Accidents happen due to lack of knowledge and awareness. Good training can also improve long term facility cost savings and time efficiency. Maintenance of the test facility is also a challenge because once a facility is up and running, down time can be costly. Scheduling maintenance around workloads will ultimately keep the equipment in good working order, prevent and reduce any unexpected downtime due to equipment failure. Effective planning and regular checks are the only way to ensure that downtime is not an issue. As the industry demands more capabilities for downhole tools, the need for a test facility that can validate to the same criteria will grow. Doing this safely will always be complex but it is a priority. Author: Jenny Bentley
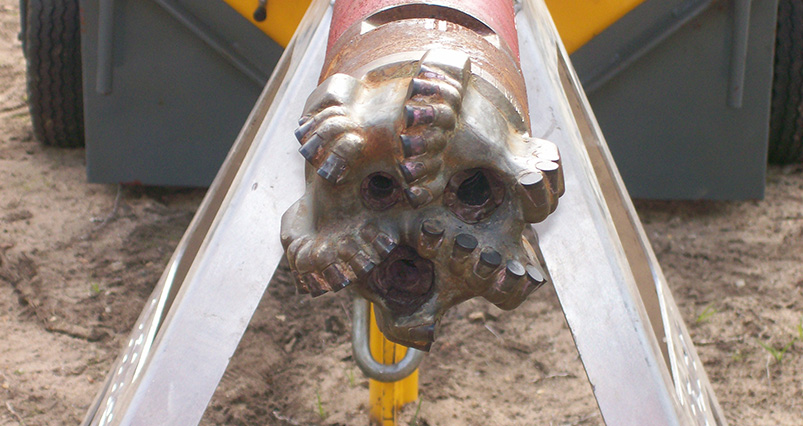
1505822400000
The Importance Of Near Bit Sensors For Coiled Tubing Drilling
There is a reason why the drivers of 18-wheeler trucks are seated at the front of the vehicle. It’s so the drivers can steer the vehicle accurately between the lanes. In directional drilling, sensors are usually spaced back from the bit because of the motor and the extra magnetic spacing required to avoid interference. This is the equivalent of steering the truck from the back of the vehicle and the results are predictable. If you consider a sensor that is 45ft back from the bit and an ROP of 15ft/hour, it will take 3 hours for the sensor to be at the same depth that the bit was. By which time another 45ft of well will have been drilled. If the trajectory is on target, no problem, you can probably predict where the new bit position is. If the measurement shows that the trajectory is off target, then not only does that error have to be corrected, there are also three hours of inaccurate drilling to correct. The result is a well trajectory that is all over the place. In thick reservoirs, this may not be so much of a problem but not all reservoirs are like this. In many cases there is one layer that is most productive and any time drilling outside that layer is wasted drilling effort. Even in thick zone shale wells, one layer will represent the sweet spot and drilling outside this layer might mean that a frac stage is not productive, simply because that stage was drilled off target. You could lose half of your productive wellbore by exiting below the zone, correcting and then overshooting before repeating the cycle again until you hit the target. Figure 1. Navigating the sweet spot A good directional driller in a known formation will reduce this error, and that is what makes them so valuable, but even a good directional driller can get caught out before they have established how a BHA responds in a particular formation. The nearer the sensor is to the bit, the faster the information is relayed back to the driller or the automatic steering algorithm. This results in a more accurate wellbore because off-target drilling can be detected earlier. Some BHA’s include near bit sensors with short hop telemetry to solve the problem. There are other gyro steering sensors that can be located just above the motor in a directional BHA because they are not affected by magnetic interference. There are also measurements that will give a reading as the bit passes the interface between one formation and another. Another feature that can help reduce directional drilling errors is the speed at which the downhole data is transmitted to surface. Faster telemetry means that more data can be sent in the same time or it is received earlier. Both of which help the directional driller to respond. In modern coiled tubing drilling systems, the telemetry is over wireline which gives the driller almost instantaneous feedback about tool position, weights, torque and vibration. The benefit of this can be seen from a real-world example shown below. This well had a target zone of 4m in thickness, and the wellbore had to be maintained within this envelope (dotted lines). The original wellbore had been drilled with a conventional directional drilling BHA and is shown in blue. The red line shows the path drilled with a CTD BHA after performing an openhole sidetrack. Allowing for the fact that the two well bores are in a different plane, it can still clearly be seen that the wellbore drilled with CTD maintained its trajectory much more accurately than the original wellbore drilled with a conventional mud pulse BHA and with with sensors further back from the bit. Figure 2. Real-world example of navigating the sweet spot Note: Not all sensors suffer from distance to bit, the directional and logging sensors are but WOB and TOB, in particular respond to what is happening at the bit even though the sensors are actually remote from the bit. Author: Toni Miszewski
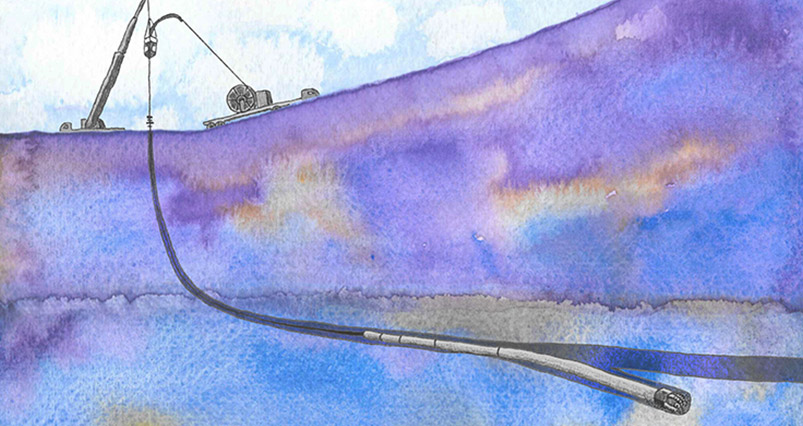
1501070400000
How To Drill An Openhole Sidetrack With Coiled Tubing Drilling
When planning an openhole sidetrack there are a number of choices to be made which will affect the ease and speed at which the sidetrack will be initiated. The easiest orientation to choose is to exit from the low-side of the wellbore as this allows the maximum weight from the BHA and toolstring to assist in the bit’s side cutting. Additionally, the more deviated the wellbore is at the sidetrack point, the more that weight will help. Choosing a high AKO setting for the bent housing motor will increase side-cutting to further aid exit from the moterbore, however the intended sidetrack may require a lower AKO setting so there is a trade-off between sidetrack speed and the need for an extra trip to adjust the bend in this case. Particularly when using low AKO settings it helps if there is a dogleg in the opposite direction at the sidetrack point. It is also important to calculate the length of time-drilled sidetrack that will be required for the entire bit to exit the motherbore, and to add a suitable safety factor to this to account for differences in dogleg and to make sure that full WOB is not applied too soon, breaking the ‘ledge’ and putting you back hours in operational time. General guidelines relating to the best practises in time-drilling an openhole sidetrack from a deviated wellbore have existed for a long time and differ very little from source to source. With these as a guideline, the real-time data available from e-coil conveyed drilling assemblies, in addition to the accuracy of electric-drive CT injectors, removes a lot of the guesswork from the process and allows for a faster, more reliable sidetrack initiation. While creating the initial trough for the sidetrack, realtime WOB allows for CT stretch to be accounted for by locating the zero point between compression and tension. This makes it easier to create a good quality starting ‘ledge’ at the downhole end of the trough. When continuing with time-drilling the sidetrack it is easy to see the bit cutting by observing the WOB and torque slowly reduce while lateral vibration slowly increases. This, coupled with the small adjustment capability of an electric-drive injector means that the bit can be advanced as required rather than basing it on timing alone; it also has the extra safety of not over-shooting the advancement interval. Illustration by Harriet Williams Author: Joe Heaton
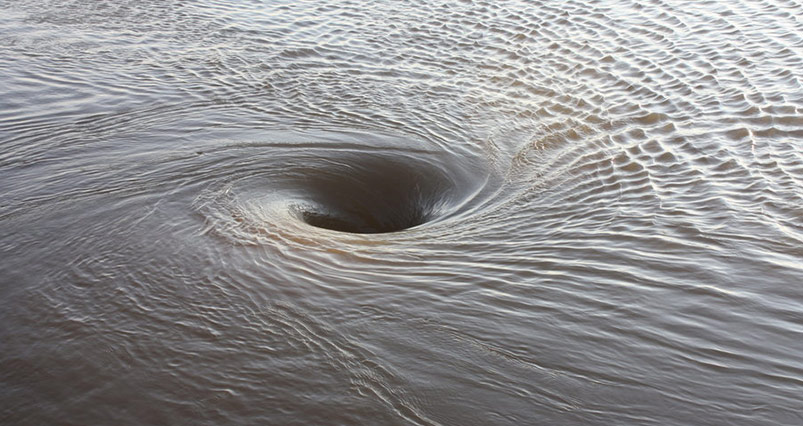
1500638400000
Combating Lost Circulation Using Underbalanced Drilling & CTD
On November 20, 1980, Leonce Viator Jr, accompanied by his nephew, took his boat out onto Lake Peigneur in Louisiana to catch some catfish. At the same time on a nearby drilling rig, the crew noticed that the drill bit had just become stuck. This was a sign that they had just accidentally drilled into a salt mine of the Diamond Crystal Salt company. The rig started to tilt and was eventually consumed into the ground. Eleven barges and a tugboat were sucked into the ensuing vortex. Leonce just managed to make it to shore and the last thing he saw was his boat and the tree it was tied to, disappearing down the vortex. This was an extreme example of a lost circulation event when drilling. Not all lost circulation situations are as dramatic, or environmentally questionable, but they can still cause problems with drilling, non-productive time, increased costs, damage to the reservoir and delay in bringing the well on line after drilling. Lost circulation can be either as a result of the properties of the formation or it might be induced by drilling conditions or practices. Ultimately, although the cause might be different the result is the same: Drilling fluid which would normally flow up the annulus gets lost to the formation, lost circulation material (LCM) may need to be added to stem the flow and this can in turn permanently damage part of the reservoir that might have turned out to be the most productive zone of the reservoir due to its high permeability. There is a risk of differential sticking and also of a blowout as the pressure in the annulus drops. Natural fractures or low pressure zones with high permeability are both risk factors for lost circulation. Lost circulation issues can occur all over the world but some examples for the Austin Chalk, Buda and Georgetown reservoirs in South Texas, USA, can be found in several SPE papers. Another way in which the formation can become fractured is from the use of excessive mud weights, run-in-hole speeds or annular build-up of drill cuttings, all of which can cause the pressure in the borehole to exceed the fracture gradient of the formation. This is particularly important when drilling overbalanced because the mud column is weighted up to contain formation pressure so the baseline pressure is already high. With underbalanced drilling, the main lost circulation problems are avoided because the pressure in the wellbore is lower than that of the formation. Differential sticking is no longer a risk and if a zone starts to produce, the flow can be controlled by the choke at surface and the produced fluids can be handled with the flow back equipment that is a part and parcel of an underbalanced drilling set-up. Coiled tubing drilling is the only way to achieve underbalanced conditions at all times when drilling, so is an ideal technology to consider for projects where lost circulation is a problem. To complete the initial story, Lake Peigneur changed from being a fresh water lake, 11ft deep, to being a salt water lake, up to 1300ft deep. Leonce never went fishing on Lake Peigneur again. Author: Toni Miszewski
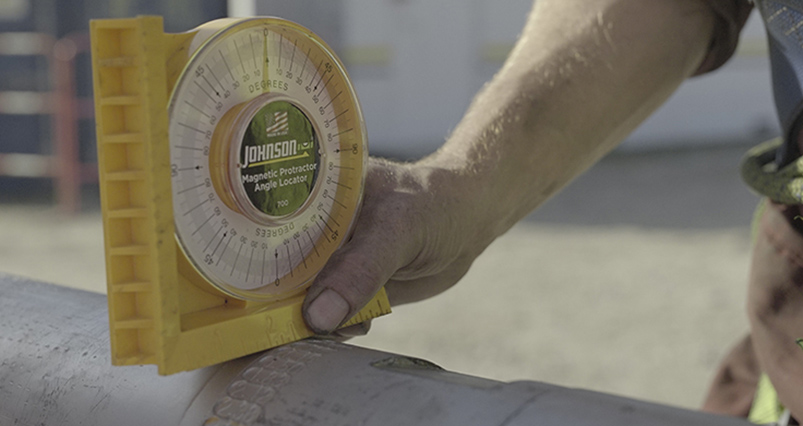
1499860800000
How Do You Drill A Directional Well With Coiled Tubing?
A Directional Coiled Tubing Drilling (DCTD) BHA is made up of the following components: bit, motor/turbine with bent housing, sensor module, orienter, electric release, cablehead, and tubing end connector. There are other optional modules that can be added to the BHA for different operations and environments, for example, an electric circulation valve, gyro and CCLs. To drill a well directionally on coil, the two most important components are the orienter and the motor/turbine with bent housing. Directional drilling on CT utilises the conventional bent housing or bent sub motor technique. The bent sub acts as a pivot and the bit is pushed sideways along the hole. The side force on the bit changes the hole direction and inclination as you drill ahead. The usual bend is set between 0°- 3°. The direction the bit is angled towards, is called the toolface. By orienting the toolface you can drill a directional profile: hold a set toolface and the BHA will drill a turn or build section, continuously rotate the toolface and the BHA will drill a straight, tangent section. Holding a toolface while drilling ahead is referred to as 'sliding' and continuously rotating the toolface while drilling ahead is referred to as 'rotating'. This information is captured on the Directional Driller's slide sheet. The only option for orienting the toolface while drilling on coiled tubing is to use a downhole orienter. The best option is to use a fully electrical orienter that can orient the toolface at any angle from 0-360° based on commands sent from the Directional Driller, operating the tool at surface. In addition to being fully electrical, the orienter should have the capability for continuous rotation to drill straight hole sections. Some orienting tools on the market cannot be continuously rotated and therefore cause tortuosity (in other words "wavy" wellbore) which reduces lateral length, reservoir contact and well productivity. Author: Adam Miszewski
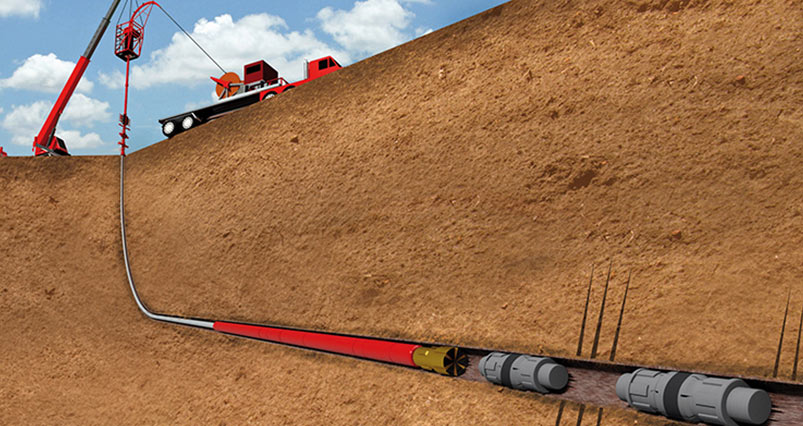
1499256000000
Why A Milling Monitoring Tool Is A Good Friend To Have On Plug Mill-Out Operations
Frac plug milling is the final step on the journey to completing a shale producer. Return on the investment that has gone in to drilling the well and pumping the frac is contingent upon completing this step successfully. A Milling Monitoring Tool can be indispensable in execution of this critical operation. Here’s how it can help. To avoid fluid loss to the new perforations, the plugs optimally should be milled while the pressure inside the completion is kept below that of the formation. Whether this underbalance is achieved with a single phase light fluid, or by injecting gas, continual monitoring of downhole pressure delivers assurance that optimal conditions are maintained throughout the milling operation. Deployed on e-coil, the MMT’s wired telemetry system is unaffected by gasified fluids and the high data rate permits second by second control of milling parameters to optimise performance. Both internal and external pressure are monitored, giving the ability to observe lifetime trends in the motor’s performance, empowering the operator to proactively pull an aged motor once readings indicate that it is about to become a dead horse. Milling often takes place along extended reach laterals – monitoring injector weight alone gives the CT operator very poor knowledge of the weight applied at the bit, yet this parameter is critical to milling the plug efficiently. It is necessary to set down enough weight to get the mill to bite. Too much weight and the motor will stall, too little and the plug will work harden, the mill will polish and penetration will be minimal. Milling with gasified fluids add presents additional problems. It is easier to get into a stall as there is almost no positive torque response as the bit slows down. When the stall happens, it takes much longer to detect at surface, as the pressure response at the gauge is softened by the gas phase. Thirdly, thanks to the compression in the gas phase during the stall, the energy released when the bit becomes unstuck can overspeed the motor and junk it prematurely. The MMT puts the coil operator back in touch with what is happening down hole. Second by second readout of Weight on bit and Torque means that the mill can be kept optimally engaged with the plug, while real time vibration indication enables stalls to be detected – and most importantly remedied, before damaging amounts of energy accumulate in the CT. To sum up, an MMT can help to maintain the pristine quality of the new frac, can speed up the plug milling time and maximise the life of the milling motor. It’s a good friend to have on the journey. Author: Richard Stevens
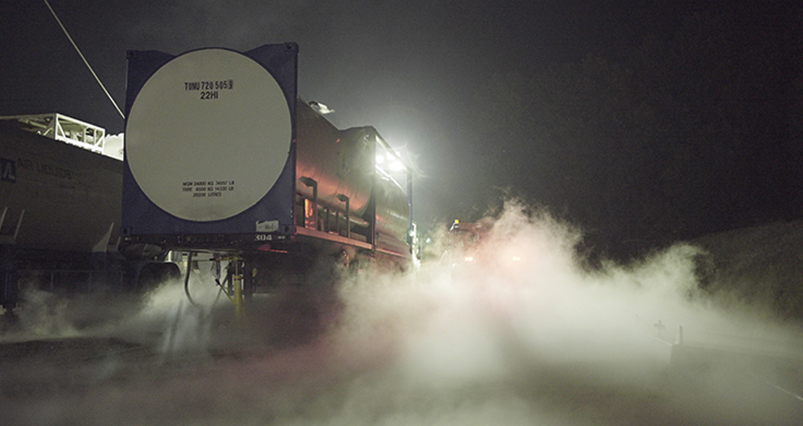
1498651200000
How To Drill Faster With Coiled Tubing
We often get asked what is the effect of underbalance on ROP, so it’s nice to have a real-life example which shows the difference it can make. In this example, the ROP increases by almost 2.5x. The change is dramatic and happens as soon as the pressure in the borehole drops to the formation pressure. You can also see an increase in improvement as the pressure in the wellbore drops. In this particular case, the pressure drop was created by adding nitrogen but there are also situations where the same difference in pressure could be achieved just by changing the mud weight. Figure 1 - Effect of Underbalance on ROP for a single BHA run The mechanism for this is quite straight forward and is controlled by the wellbore to formation differential pressure (i.e. amount of pressure in the wellbore over or under the formation pressure). When the well is in an overbalanced state, the well pressure is higher than the formation pressure, and the pressure exerted by the drilling fluid is holding down the cuttings. It also acts like a confining pressure on the formation, making it harder to drill. The power at the drill bit is fighting the overbalance differential pressure. When drilling underbalanced, there is no hold-down effect on the cuttings. The underbalance pressure is now working in the opposite direction and the formation pressure helps dislodge cuttings, increasing ROP and bit life. The power at the drill bit is being helped by the underbalance differential pressure. is ideally suited to underbalanced drilling (UBD) through the continuous pipe and inherent pressure control capabilities. This coupled with fast tripping times and lack of connections means that CTD can be a very fast drilling method. Based on this example, it’s interesting to consider what would be the effect on drilling productivity if all wells were drilled underbalanced and had a similar improvement. Compared to a rig drilling overbalanced, an underbalanced rig could drill the same number of wells in a year. If both rigs started in January the underbalanced rig would be finished in June and have 6 months of revenue generating production before the overbalanced rig had finished drilling its last well! Author: Toni Miszewski
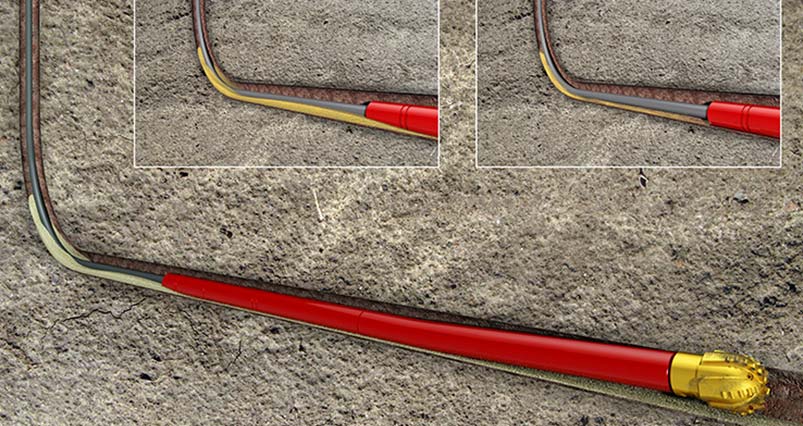
1498132800000
Introduction to CTD Wellbore Hydraulic Modelling - Part Two
In my last blog post, I introduced the principles of hydraulic modelling for coiled tubing drilling. I left you with this beautiful chart, depicting the pressure distribution throughout the hydraulic circuit for a specific set of operating conditions. Figure 1 - Pressure Profile This chart shows us, for a given depth point in a particular well, for a particular pumping rate, through given CT and BHA, the pressures at every point in the system. Points of particular interest are: Bottom hole circulating pressure (BHCP) If we were not circulating, this would be simply the hydrostatic head of the fluid in the wellbore. To that hydrostatic component, we add the frictional pressure due to the velocity of the fluid moving up the wellbore and any choke pressure applied at surface. If we are drilling conventionally, we will seek to maintain this BHCP a little above the formation pressure to maintain well control. If this pressure is allowed to rise too far above the formation pressure, we may end up unintentionally fracturing the formation and losing circulation. As we are speaking in the context of coiled tubing drilling, it is more likely that we will want to maintain this pressure a little below formation pressure, allow the formation to produce while drilling and keep the matrix uncontaminated by drilling fines. Guide arch pressure When coiled tubing comes off the reel, over the guide arch and down the hole, it straightens, bends and straightens again. This represents extreme plastic strain of the material and leads to failure of the material after a small number of cycles. It is well understood that the exact number of cycles before fatigue failure is strongly dependent on the pressure inside the CT when it is plastically bent. The dependency is non linear, such that an increase in pressure of 1000 psi from 2000 to 3000 psi can more than halve the fatigue life of the coil. It is therefore of vital importance in estimating the cost of a proposed job, to know what the pressure at the guide arch is likely to be, in order that the percentage use of the coil’s fatigue life may be accurately costed. Pump Pressure Ultimately it is the rig pumps that maintain the circulation that causes the interesting pressure profile that we have seen above. Knowing the required pump pressure is vital in specifying the correct equipment for the job. Once a pump is specified, knowing the pressure and flow rates expected throughout the job enables a total fuel burn to be estimated, further refining the job’s economic model. Informative and useful though this undoubtedly is, we have to recall that the chart in figure 1 is correct for only one flow rate (or one set of flow rates if we are pumping a 2 phase mix). Increase the liquid rate or reduce the N2 rate and the picture changes. How much more useful if we could combine the information in figure 1 with sensitivity to flow rates. For the moment, let’s assume that we are going to maintain the liquid pump rate at, say, 40 USGPM. We can vary the N2 injection rate in 6 steps between zero and 1000 scfm. That gives us six flow rate specific versions of figure 1. All six are presented below as figure 2. Figure 2 - Pressure profiles for varing N2 rate at fixed liquid rate of 40 USGPM If we study the results in figure 2 we see a trend in BHCP with N2 rate. Let’s plot BHCP vs N2 rate for this set of results. Figure 3 - BHCP vs N2 rate for results in figure 2 If we continue to study the results in figure 2, it is clear that there is also a trend in guide arch pressure. We can plot that trend on the same set of axes. Figure 4 - Variation of BHCP and Guide arch pressure with N2 rate for fixed liquid rate Now we can repeat the process for a different fixed liquid flow rate. Let’s say 60 USGPM this time. Here are the 6 sets of pressure curves. Figure 5 - Pressure profiles for varing N2 rate at fixed liquid rate of 60 USGPM Again, we can see the trends in BHCP and guide arch pressure. Just like before, we can add the trends to our XY plot. Figure 6 - Combined plots of BHCP and Guide Arch Pressure for 2 liquid flow rates I’m sure you can see where this is going. We can add curves for as many liquid flow rates as we feel are necessary to present a clear picture. What we get as a result is a single sheet that tells us at a glance what combination of pump rates we need to maintain to get us a particular BHCP. Once we have identified an operating point, all we need to do is track vertically until we hit the corresponding guide arch pressure line, then read off horizontally what our guide arch pressure will be at our chosen operating point. An example is shown in figure 6. A combination of 60 USGPM of liquid and 800 SCFM of N2 gives us a BHCP of 550 psi. Reading vertically up until we hit the green dashed guide arch pressure line corresponding to that liquid flow rate, then across, we see that the guide arch pressure at that operating point is 1400 PSI. We can add a horizontal (pressure) cursor to indicate the pore pressure in the formation we are drilling, and we can add limit lines for our mud motor. We can extend the principle we applied to BHCP and Guide arch pressure above, to bring out pump pressure and display it in the same way. Figure 7 - Combined plots of BHCP and Guide Arch Pressure for 2 liquid flow rates You’ll agree that the chart is looking a little crowded now. However richness of information delivered in a single image makes perseverance worthwhile. The observant reader will notice something about the BHCP lines. They vary in thickness. We use this to convey one more vital piece of information gleaned from the hydraulic model – hole cleaning. Exactly how we do this is the subject of a future blog. Author: Richard Stevens
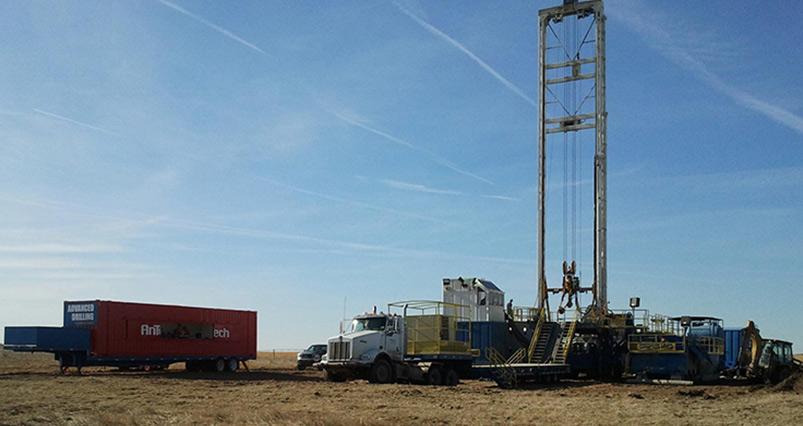
1493208000000
Selecting a Coiled Tubing Unit for CTD: Part Two
Following on from , this article continues to explore the areas required to select a suitable CT unit for Coiled Tubing Drilling operations. Price & PerformancePrice is the obvious one! However, the cost is never the only consideration and care should be taken to review the CTU provider’s performance history. Furthermore, you should look at their accident and incident records and their safety culture. CTD should always be considered as a technology that offers a high return on investment but this does not necessarily mean the lowest upfront cost. A CTU provider may choose to charge in a number of ways. This could include a basic day rate with itemised charges for elements such as coil fatigue, mobilisation for example. Both the Operator and the CTU provider should be fully aware of exactly how the CT is going to be used i.e. the unique drilling practices associated with CTD. Pressure Control Equipment (PCE)Careful consideration must be given to the PCE and its pressure rating. A CTU provider may work on wells under a variety of pressures and so should have a selection of BOP stacks and other PCE available. The PCE must be selected in accordance with the expected well pressures and operations. All potential well control scenarios should be considered in a risk assessment along with the selected PCE. Data RequirementsThe quantity of data that it is possible to capture from a CTD project is vast. Serious consideration needs to be given to what information you would like to capture and how this can be achieved with the chosen CTU provider. Your CTD BHA provider should be able to provide the requested data acquisition services for all the services onsite if required. Choosing a CTU that has advanced data acquisition and transmission systems will facilitate displaying data to the interested parties whether you use the BHA provider as a hub or the CTU provider. Completion TypeThe operations that are carried out before and after drilling an open-hole section with coiled tubing can have extremely significant cost impacts. If there is a requirement to pull completion tubing before drilling and/or to run completion tubulars after drilling, then a workover unit may need to be used. This inevitably adds extra mobilisation costs and rig up/down time. The most effective way to carry out operations on wells like this is to utilise a hybrid CT unit. Whilst these units are capable of running pipe as well as coiled tubing, they are quite rare. However, if utilised, they can have significant, positive impacts on the economics of the well. Author: Adam Miszewski
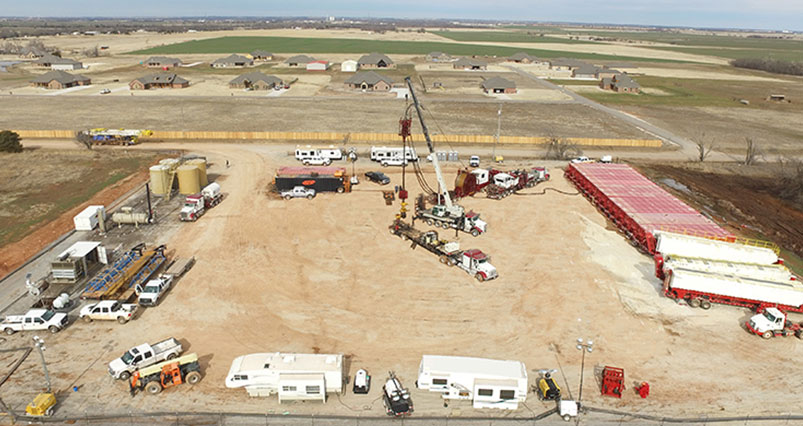
1492603200000
Selecting a Coiled Tubing Unit for CTD: Part One
In order to select an appropriate coiled tubing unit (CTU) and CTU provider for CTD operations, a few key steps must be taken, with focus on specific criteria to ensure a successful operation is conducted. Initial feasibility studyAll CTD projects must carry out a feasibility study before progressing. An initial trajectory plan must be made and the well modelled to assess the technical requirements of the CTU. The computational modelling carried out in the feasibility study defines the coil size, trajectory and lateral length trade-offs. The operator should weigh these factors against their impact on the well objectives. Once this decision has been made, the required coil specification to carry out the job successfully can be selected. This will be the first initial filter for coiled tubing unit selection. eCoil SpecificationThe feasibility study will have provided the basic coiled tubing specification, however, further detailed engineering planning will have to be carried out to determine the exact coiled tubing specification. This process will involve assessing what is required against what is actually available. At this point in time, consideration should also be given to the type of well fluids to be used, for example the H2S content. Having determined the size of the coil, the BHA provider will then specify which type of wireline is to be used inside the coiled tubing. Most BHAs on the market require heptacable and it is important that the correct specifications are outlined to the coiled tubing provider. Doing so early in the initial planning stages ensures the availability of the correct wireline and CTU conversion equipment. CT Unit Capacity and ControlThe CT unit must be able to hold the required length of the coil specified to carry out the job. This includes any additional length needed to carry out fatigue and slack management cut backs. Coiled tubing drilling can require fine adjustment of the coiled tubing and, therefore, the optimum CT units are those with a fully electric control system for their injectors or units with electric over hydraulic. Alternative control systems can be used but are not optimal. The injector should be capable of providing a suitable overpull downhole in the event of a stuck pipe. Deployment MethodThere are three main types of deployment method: injector and PCE stack up suspended from a crane, tower system, and a mast based system. There are advantages and disadvantages to each of these. The type of deployment method to be implemented can have a significant impact on your CTU selection. Author: Adam Miszewski
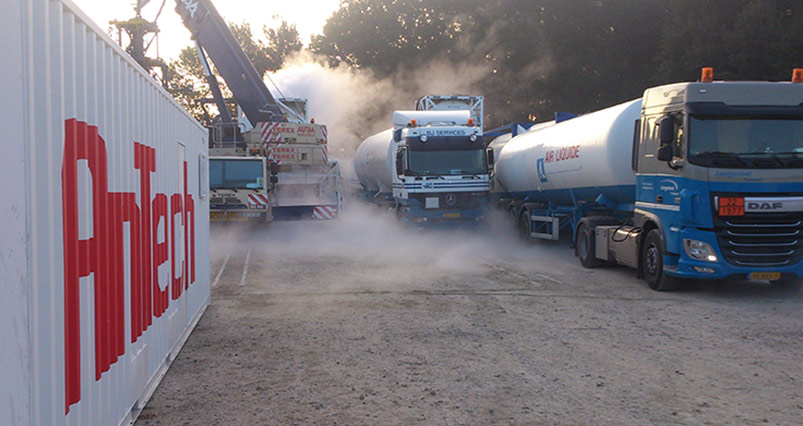
1490788800000
Introduction To CTD Wellbore Hydraulic Modelling - Part One
In the last article I introduced the importance of modelling in the context of Coiled Tubing Drilling and explored how a fit-for-purpose tubing forces model can inform engineers about the weight that can be transferred to the bit and the likely length of any lateral that can be drilled, long before any trucks hit the road. In this article I shall take the same approach with hydraulics modelling. Firstly, lets recap why hydraulics matter. Drilling produces cuttings If we are drilling a 4-1/2 inch diameter hole with an ROP of 20 ft/hour then we are going to be producing around 320 cubic inches of cuttings per hour. To avoid burying the BHA and creating a cuttings bed around the Coiled Tubing, these cuttings need to be conveyed out of the hole. The cuttings are conveyed along the lateral and up the annulus by the same mechanisms that transport sediment along a river bed. The important thing in our case is that, unlike the river, we must not allow the cuttings to settle at any point along their journey. The mechanisms of particle transport in fluid media are well understood, and given knowledge of the wellbore and completion diameters it is straightforward to predict the annular velocities that will need to be present at all parts of the wellbore in order to keep it clean. Pressure matters downhole We need to keep the well under control while we are drilling it. In conventional drilling terms, keeping the well under control means maintaining a hydrostatic column in the wellbore that just over matches the pore pressure in the formation being drilled so that the hydrocarbon stays put. In Coiled Tubing terms, the definition of ‘under control’ is stretched to encompass the useful condition of having the hole slightly underbalanced so that the reservoir produces while the hole is being drilled. But we mustn’t let the pressure in the hole get too low, or borehole caving will take place, where the differential pressure between the formation and the borehole causes chunks of the borehole wall to break off, potentially leading to pack-off of the BHA.Equally, we must not allow the borehole pressure to exceed the fracture pressure of the formation, or we will incur losses and contaminate the formation. Pressure matters at surface too The pressure required at the output of the mud pump is the sum of all the pressure losses in the fluid circuit. On a CT job, these can be lumped as: Pressure drop across the CTPressure drop across the BHAPressure drop across the annulusPressure drop across the choke Clearly it will be useful to be able to predict the pump pressure, in order to be sure of turning up at the well site with correctly sized kit. It is also useful to be able to predict the Injector Guide Arch Pressure. That is, the pressure inside the CT at the position where it enters the injector. This is because coiled tubing suffers from low cycle fatigue due to plastic bending as it comes off the reel and over the guide arch. It turns out that the fatigue life of the coil is strongly dependent on guide arch pressure, so having a handle on expected guide arch pressure ahead of the job is important in determining what proportion of the CT’s life cost should be defrayed against the job. So having identified how important it is to have a hydraulic model, how do we go about creating one? Figure 1 shows a simple horizontal wellbore being drilled with Coiled Tubing. Fluid flows down the inside of the CT, through the BHA, which will likely have a smaller flow diameter than the CT itself, through the mud motor, through the bit nozzles and across the bit face where cuttings get entrained. Finally, the fluid, now carrying cuttings, flows along the horizontal section of the annulus, up round the build and up the vertical section to the surface. Figure 1 - A simple horizontal wellbore being drilled with CT Figure 2 - 'U' tube representation of CTD wellbore hydraulics At the start of the modelling process we make an assumption. We assume that we can make a fairly accurate guess at the absolute pressure at one point in the system. That point is the fluid exit at the top of the annulus. In the simplest case we can assume the (gauge) pressure at this point is zero. Now let’s consider a point 50 ft down the annulus. At this point we can confidently say that the hydrostatic component of absolute pressure is 0.43 psi/ft x 50 ft = 21.5 psi higher that it was at our starting point (assuming we are pumping water). We can also find expressions for the frictional pressure loss over this length of annulus in terms of pump rate, fluid viscosity, tubing sizes and annular wall roughness. To learn more, start by googling ‘Darcy Weisbach’. So we can come up with a good guess at absolute pressure at a point 50 ft down the annulus. We can repeat this procedure at 50ft steps all the way down the annulus, round the bend and along the horizontal, remembering that in the build and horizontal sections, a 50 ft change in measured depth results in a smaller change in vertical depth. (It is vertical depth that causes the hydrostatic component of pressure). By the time we reach the annulus side of the bit, we have our estimate of Bottom Hole Circulating Pressure.Well validated expressions are also available for pressure drop across a nozzle, giving us the pressure drop at the bottom end of the mud motor. We typically model the motor as a fixed pressure drop. Motor manufacturers publish data sheets which reveal what a sensible value of pressure drop would be for a particular motor. Figure 3, an example for a 3-1/8" mud motor from Toro Downhole Tools, suggests that 350 PSI would be a sensible figure to use for that motor. Figure 3 - Performance curves for a typical 3-1/8" mud motor, image courtesy of Toro Downhole Tools That brings us to the bottom of (the remainder of) the BHA. Depending on the manufacturer and purpose, the flow path through the BHA may be annular or circular in section. An appropriate form of the Darcy Weisbach equation can be formulated to predict the pressure drop across the BHA. The CT between the BHA and the surface can be tackled in a similar, step by step manner to the annulus, remembering that the flow section is now circular, and that the upward calculation will continue, in steps, above 0 ft MD, to provide results for the length of CT still on the reel. The result of these calculations, which for reasons of efficiency are best performed on a computer, is conveniently expressed in a graph like the one in Figure 4 below. Figure 4 - Typical hydraulic circuit pressures for CT Drilling Figure 4 demonstrates how, armed with details of the hole trajectory, critical BHA dimensions, casing diameters, tubing sizes etc., an engineer sitting at a desk can crunch the numbers and generate a wealth of information about a prospective job, and make an assessment about that job, without incurring the expense of trying it for real.Figure 4 tells us the pressure distribution through the hydraulic system. We still need to work out fluid velocities, to be certain of effective hole cleaning.We also need to remember that, while the picture in figure 4 relates to a single flow rate of fluid at a single bit position, to be truly useful we need a representation that addresses an entire drilled section and displays sensitivity to pump rates. We’ll tackle these items in the next article. Author: Richard Stevens
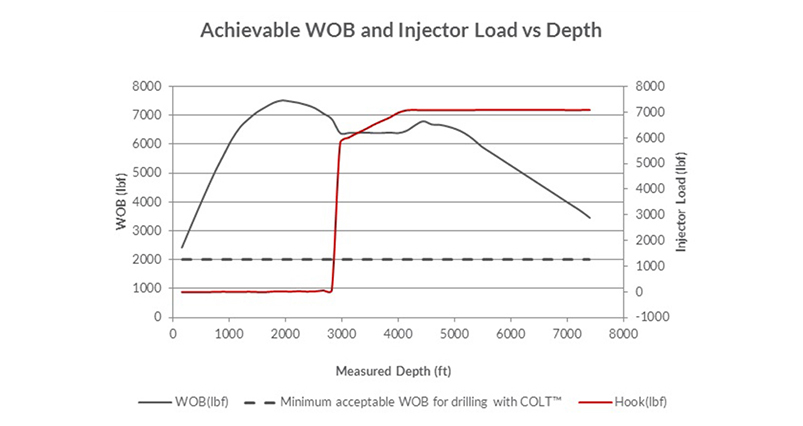
1487332800000
How Computational Modelling Contributes To Planning A Successful CTD Campaign
As anyone with a succession of successfully executed Coiled Tubing Drilling jobs under their belt might tell you, ‘Coiled Tubing Drilling is Great! It’s a lightweight, small footprint, highly flexible environmentally friendly operation, with a low staff count and a small fuel burn. Tripping speeds are fantastic, pressure control is excellent and thanks to the inherent ability to drill underbalanced, reservoir damage is unheard of.’ And yet, depending on the company you keep, you may be equally likely to run in to someone who will tell you, “It’s a fiddly, trouble-prone operation. You can’t get weight to the bit, you can’t clean the hole, the tools get stuck and fatigue breaks the CT. It is a fact that there are some drilling jobs totally unsuited to CTD, and those who hold the latter view are likely to be those who have been burned trying to use CTD in situations where it has no hope of delivering on its claimed advantages. The way to avoid being burned is to thoroughly appraise the proposed job beforehand, including full computational modelling of the operation. It is the computational modelling that is the subject of this series of blog articles that will be posted over the coming weeks. The success of a CTD project depends on three fundamentals: 1) Tubing Forces To be successful it is necessary to be able to convey the bit to the bottom of the hole with enough push left over to engage the bit with the formation and maintain positive Weight On Bit. 2) Hydraulics Success depends on the ability to circulate enough fluid through the coil and the BHA to power the mud motor and clean the hole while maintaining the bottom hole circulating pressure in a tightly defined window – high enough to prevent the hole collapsing yet low enough to avoid formation skin damage through fluid invasion. 3) Fatigue Management Every time a length of CT comes off the reel, over the guide arch and in to the hole it experiences 3 episodes of plastic deformation. Repeated plastic deformation can rapidly add up to catastrophic failure. The number of cycles to failure turns out to be strongly dependent on the pressure inside the CT when the plastic deformation takes place. Success depends on managing this fatigue by tracking every motion of a reel of CT throughout its life, in conjunction with the pressure present so that the coil can be removed from service before it has accumulated enough fatigue cycles to fail catastrophically. Virtual jobs can be modelled in advance, and the results combined with the history of a candidate coil to determine whether a particular coil can be used for a project without exceeding its fatigue life. All three of these fundamentals can be modelled, using fit for purpose software such as AnTech’s ATLAS™ software. The results of modelling give an unambiguous indication of whether or not a proposed project is a good candidate for CTD. For the remainder of this article we will concentrate on tubing forces modelling. The other fundamentals will be covered in future articles. Tubing forces modelling Imagine a vertical hole in which a Drilling BHA is deployed on Coiled Tubing. When the bit reaches the bottom of the hole (fig 1a), a first order approximation of the available weight on bit can be made by adding the weight of the BHA to the product of the CT’s weight per foot and the depth of the hole. The CT is a tall slender column, and the approximation above holds true until the column buckles. Once the column buckles, there is a frictional interaction between the outer walls of the CT and the inner walls of the hole (fig 1b). The load level at which buckling commences is a well understood function of the CT’s dimensions and material stiffness, and the frictional forces due to the interaction between the buckled CT and the wall are primarily a function of the difference in size between the CT and the hole it is in. Now consider that we have drilled a build to horizontal at the bottom of our vertical hole, followed by several hundred feet of lateral. When the BHA is in the lateral (Fig 1c), it is still the weight of the CT in the vertical section that is the motive force propelling the BHA sideways (except in very shallow cases where the injector’s snubbing ability may be usefully used to assist); and as in the vertical case, this force is potentially moderated by buckling. Additionally, frictional forces due to the weight of the BHA and the CT on the bottom of the lateral act to oppose the driving force. As the lateral gets longer a point will be reached when these frictional forces outweigh the propelling force and the hole will cease to progress. If you are an engineer putting a project together, what you want to know is whether or not you will be able to generate sufficient WOB to drill at all points along the proposed wellbore’s trajectory. ATLAS™ is designed to deliver just that. All it requires is a trajectory and the details of any existing completion that the tool string will be running through, details of the BHA and CT, and the density and viscosity of the drilling fluid being used. ATLAS’s headline tubing forces output is a chart showing achievable WOB against measured Bit Depth. Also shown is Injector load, and a minimum WOB threshold for drilling. Figure 2. Atlas primary tubing forces output. Achievable WOB vs Depth This output enables the engineer to determine at a glance whether his chosen CT and BHA configuration will reach the end of the hole with enough available weight on bit to drill. In the case shown in figure 2, there is still 3000lb available WOB predicted at the end of the hole, so the engineer knows he is good to go. His next step will be to consider the hydraulic aspects of the job. These will be discussed in the next blog. Author: Richard Stevens
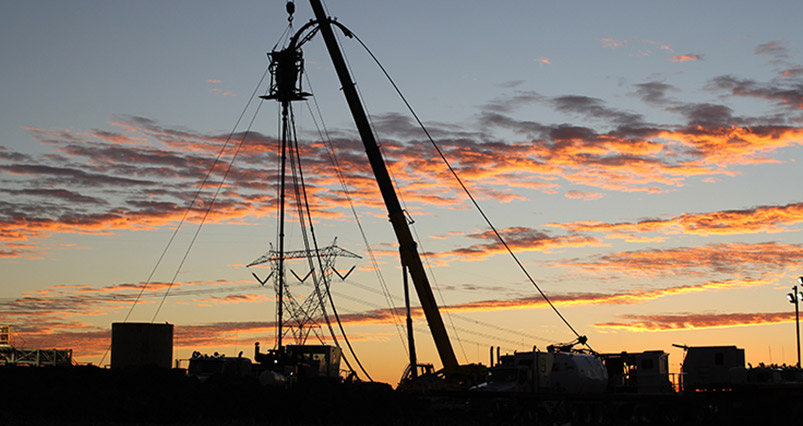
1485950400000
Jointed Pipe Versus Coiled Tubing Underbalanced Drilling
Underbalanced drilling (UBD) is defined as drilling when the effective downhole circulating pressure of the drilling fluid is equal to, or lower than, the formation pressure. There are many advantages to UBD including minimised formation damage/skin and therefore increased well productivity, increased ROP, and reduced risk of lost circulation as well as many others. The main disadvantages are additional complexity, higher risk of equipment failures, and the incompatibility of certain drilling fluids with conventional MWD systems, for example, two phase drilling fluids. Equipment Considerations Certain parts of the equipment are common across Jointed Pipe UBD and Coiled Tubing UBD operations. For example, the fluid coming back from the well still passes through a choke manifold followed by a separator. The separator can take many different forms and use different methods for separation. Both the choke and separators used are common to both Jointed Pipe UBD and Coiled Tubing UBD operations so won’t be discussed further here. Where Jointed Pipe UBD and Coiled Tubing UBD diverge is on the pressure control equipment. On conventional drilling rigs or snubbing units carrying out UBD operations, a rotating control device (RCD) becomes the primary barrier and the annular and ram preventers become secondary barriers. The RCD diverts the fluid returns from the well to the choke manifold. When drilling with single phase liquid, two phase gas-liquid fluids, or foam, the remainder of the BOP stack is not that much different from the BOP stacks used for conventional overbalanced drilling with mud. RCD’s should be considered as high pressure diverters rather than an annular preventer or BOP. Their rated working pressure is usually lowered by 50% when rotating. Another consideration for Jointed Pipe UBD is the number and location of float valves that are necessary to prevent the influx of reservoir fluids inside the drill string either when tripping or making connections. Well control is significantly simpler when carrying out UBD operations with Coiled Tubing because a coiled tubing rig up is inherently designed for underbalanced operations and pressure control. The bit is turned by a downhole motor and the pipe does not need to rotate; this allows for a standard BOP stack up, plus lubricator and a simple stripper assembly. The safest and simplest way to deploy the Coiled Tubing UBD BHA is with a suitable length of lubricator that allows the well to be shut in during rig up. Once the BHA is made up to the CT and the lubricator is made up again the well can be opened up and the BHA deployed in the safest possible manner. Although less preferable, it is also possible to deploy the BHA under pressure in two stages by using a slickline lubricator. Comparative advantages of UBCTD over conventional UBD When drilling the reservoir section of a well or sidetracking from an existing well to access another area of the reservoir, Coiled Tubing UBD usually has the edge over conventional UBD with hole sizes up to 8-1/2”. Coiled Tubing UBD has a safer, simpler and more reliable stack up. For Jointed Pipe UBD, when drilling with two phase fluids the time taken for the well to stabilise after making a connection can be prohibitive and remove a lot of the value of UBD. It is also difficult to maintain underbalance conditions when making connections as the following diagram shows. The potential of Coiled Tubing UBD Despite the obvious advantages of UBD in terms of reservoir protection and drilling jobs that have been outstanding successes, it is a technology that has not yet fulfilled its full potential. However, in parts of the world, for example Saudi Arabia, it is the advantages of Coiled Tubing technology that has made UBD be the success that it has been over many years. Author: Adam Miszewski
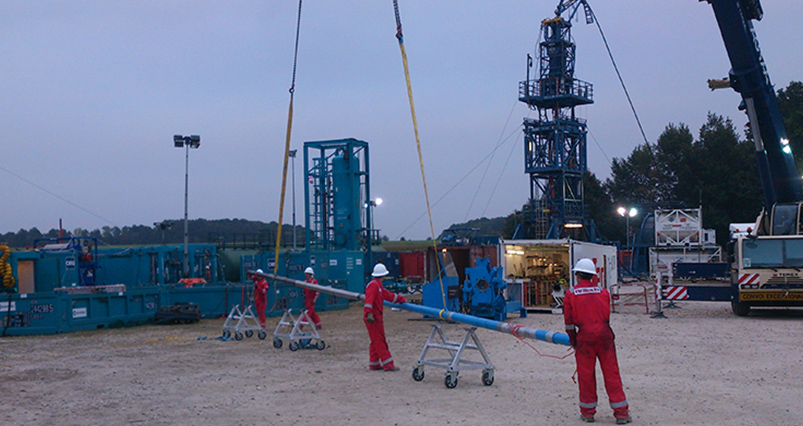
1485345600000
Benefits Of Drilling A Straight Hole With Coiled Tubing Drilling
Why a straight borehole is better than a wavy one In conventional directional drilling with a bent-sub motor, the well trajectory is drilled with a combination of sliding stages and rotating stages. This creates a curved hole and a straight hole respectively. When drilling with coiled tubing drilling, sliding is easily achieved but the coil, unlike conventional drill pipe, cannot rotate so an orienter is required to change the tool face. The importance of a continuously rotating orienter when drilling a lateral with Coiled Tubing For years, laterals drilled with coiled tubing have been drilled with a sinusoidal profile. These are created when a bent-sub motor is flipped first one way then by 180° in the horizontal plane so that the TVD is maintained and the azimuth is generally in the right direction, even if the borehole isn’t straight. The trajectory would be better described as snake-like. This way of steering comes about due to the limited rotational ability of the orienters being used. They have a rotating capability which is restricted to approximately 400 degrees of rotation in one direction before they need to be rotated back again. The continuous rotation needed to drill a straight hole is not possible.Snake-like boreholes have a detrimental effect on how far you can drill or how much weight-on-bit (WOB) can be transferred from surface. Every change in direction increases the friction experienced by the coiled tubing because of the way that the coil is pushed against the borehole wall.Straight holes constrain the coil more in line with the axis of the borehole and so more weight can be transferred to the bit and you can drill further. More modern orienters, like on AnTech’s COLT™ BHA, can rotate continuously, even if quite slowly, and can drill a straight hole and can drill the curve and the straight hole in one run. They can also be used to drill short alternating straight and curved sections effectively reducing the build-up of angle without having to pull out of the hole to change the motor bend. How much difference does it make? The reduction in performance can be quite significant. In practice, each case needs to be modelled to be able to quantify the effect using software such as AnTech’s Atlas™ software which can predict tubing forces based on the well trajectory and many other parameters. As a comparative exercise, a well was modelled as shown in the diagram above. In one case, a wavy wellbore was modelled against a straight hole and in this particular example, either twice the WOB could be applied, which will help ROP or an extra 1500ft could be drilled without going below a 2000lb WOB threshold. Author: Toni Miszewski
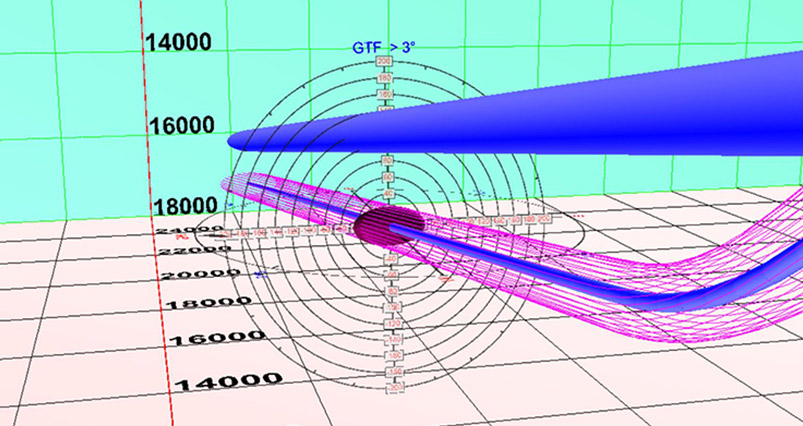
1481544000000
Leading The Way - Quality Control Of Directional Measurements
Drilling for oil began in the mid-19th century and up until the mid-20th century it was illegal to deliberately deviate from drilling vertical wells. This was due to a mixture of reasons such as avoiding collision with other wellbores and producing oil or gas which is not owned by the leaseholder. However, John Eastman changed all of this in 1930 when he was granted a patent to determine the direction and inclination of a wellbore. He achieved this using a plumb line, a compass and a camera, and simply took a photograph of the compass and plumb line while in the wellbore. Things have moved on significantly now, and we use a series of three accelerometers and three magnetometers. These are at right angles to each other, to determine how vertical the wellbore is by measuring the strength of gravity in the three directions and to measure the strength of Earth’s magnetic field, and therefore figure out which way is north. This system allows measurements to be taken while drilling, or the magnetometers can be replaced with a gyro to gain more accurate directional readings, but for this to work all operations must be shut down to reduce vibration. With a lot of wellbores being drilled in oil producing regions across the world it is becoming increasingly important to know the exact location of the wellbore underground. It is, of course, impossible to know this with 100% accuracy, so it is important to understand the errors associated with downhole measurements, and where the hole ‘could be’. Each measurement device has an associated error and when we combine all of these sources of measurement error we end up with an ellipsoid at each point in the wellbore, which represents all of the possible positions the hole could be. This ellipsoid increases in size as we drill further as our position underground is calculated from each direction, inclination and depth measurement, so the errors build up the further we go. If the wellbore is plotted with all of the possible positions, this looks like a cone with greater errors at greater distances along the hole. At AnTech we deal with this uncertainty in both the planning and the operations stage. In planning a well trajectory we also plot the error ellipses, and consider where the wellbore could actually be in relation to where we want to go. In some cases this could be the difference between reaching the target reservoir or not. While drilling we continually check that the uncertainty in our measurements fall within the error ellipse that we have specified in planning. This is done by checking the magnetic dip and total gravitational field strength, which we know in our well location, and checking the spread of azimuth data. This quality control system, alongside our continuous stream of downhole data, provides the assurance that we know where we are drilling. Author: Mark Kelly
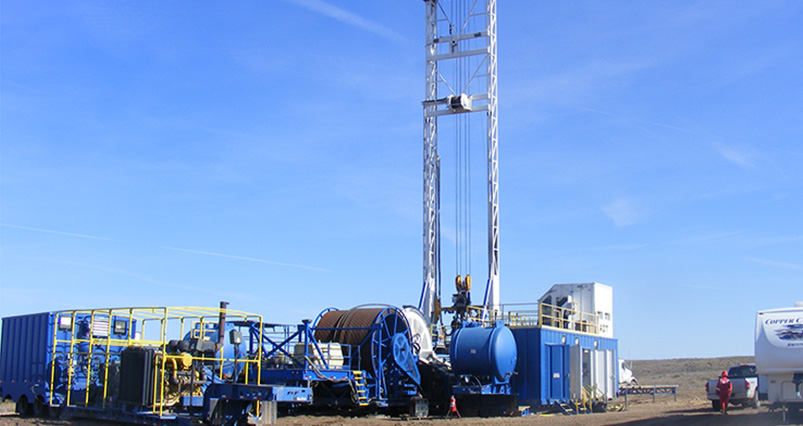
1480334400000
Why A Downhole WOB Sensor Is So Important When Drilling With CT
A direct measurement of downhole WOB is vital for accurate control of the drilling process during Coiled Tubing Drilling (CTD). Without it, motor stalls will be more frequent and directional control will be more difficult. In a vertical well with conventional jointed pipe drilling it is a reasonable assumption that the weight applied to the drill bit is directly related to the hook load at surface. This assumption starts to become less valid as soon as the well becomes deviated or horizontal because of the friction between the pipe and the wellbore. But even then, it is possible to compare upward and downward loads to estimate the friction and therefore determine WOB. With Coiled Tubing Drilling (CTD) the situation is complicated further such that the assumption that surface load is directly linked to downhole WOB definitely cannot hold. With CTD however, you definitely cannot be sure that the load you are reading at surface is the same as the load downhole. The forces which affect the transfer of weight to the bit are numerous and include: Stripper FrictionBuoyant Coil WeightPipe Friction With coil, the sliding friction is non-linear because of the CT buckling affect. This in it itself is affected by the actual WOB. If you then add the friction of the stuffing box at surface, which is dependent on the instantaneous pressure applied to it and which in turn will be affected by the variable wellhead pressure. With CTD the number of forces, their variability, non-linear nature and their interdependency means that it is impossible to determine downhole WOB from a change in surface weight. Bearing in mind that one might experience WOB’s in the order of 2000lbf for effective drilling, having an uncertainty of possibly 5 times that makes WOB determination next to impossible. The solution to the problem is to directly measure the WOB downhole in the drilling BHA. That way the measurement is taken without the influence of the measurement variables described above. All downhole WOB sensors are affected by an effect called the ballooning affect which is caused by differential pressure across the tool. The ways to correct for this are a topic for another post.Author: Toni Miszewski
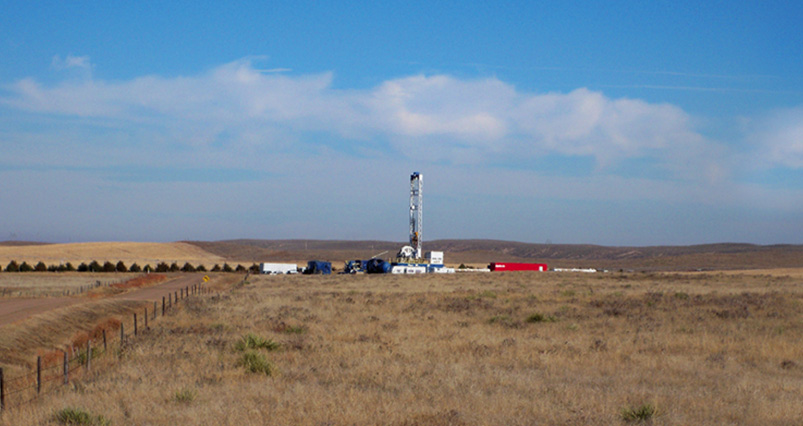
1480334400000
What Is Underbalanced Drilling & How Does It Work?
Underbalanced drilling (UBD) is when the pressure in the formation is greater than the hydrostatic pressure in the bore hole. This difference in pressure encourages the flow of oil, gas or both into the annulus and helps the well to produce. Underbalance drilling can be used in both jointed pipe and coiled tubing drilling applications. However, to achieve true underbalance, coiled tubing drilling is really the only viable method to do this. Although jointed pipe drilling can be used in underbalanced conditions, there are some features that mean it can never be classed as ‘true underbalance’. Disadvantages such as breaking equilibrium to make-and-break joints makes the process costly and time consuming along with the fact that the well is not pressure contained at all times, can decrease your safety on site. This isn’t the case with coil, drilling with coiled tubing makes it much safer for field operators and allows unexpected changes in downhole pressure, or kicks, to be safely circulated out of the system by using the choke at surface to adjust the flow back pressure. Other than safety, UBD Coiled Tubing Drilling has other advantages: Drilling is faster because the cuttings at the drill bit break away more easily.UBD allows the well to produce while drilling which means that the well owner can earn money while drilling.By monitoring the production while drilling, it is possible to navigate through the reservoir to get the best production. Differential sticking is often a common concern from clients and differential sticking and lost circulation is a common cause of stuck pipe. This happens in overbalance conditions when the mud pressure on the outside of the wall of the pipe is greater than the formation-fluid pressure. In UBD the pressure differential is the other way round so provided the mud weights are controlled correctly, differential sticking is avoided. Underbalanced conditions are created by lightening the weight of the fluid column in the well. In some cases this can be done with a lower density fluid such as fresh water or diesel. In other cases, it will be necessary to add a gaseous fluid such as air or nitrogen to achieve the required underbalance. Author: Clare Miszewska-Hall
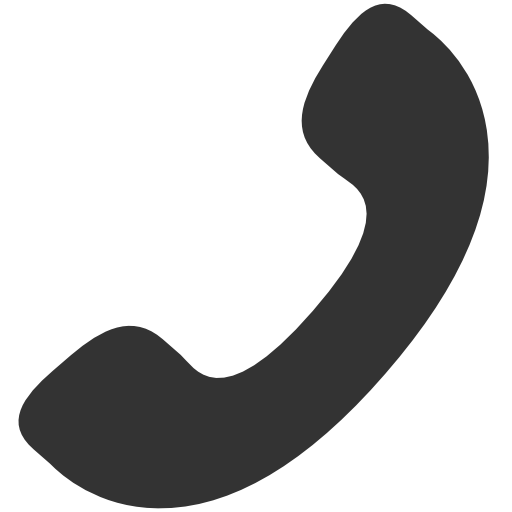
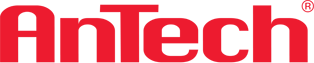
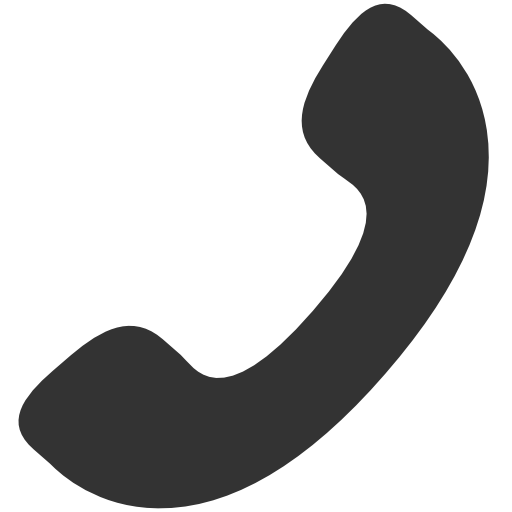

+44 1392 933 100 | +1 800-868-1562